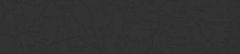
Topics Explored: Nitrogen Cycle, Transformation of Energy, Trophic Levels, Food Chains, Food Webs, Population Interactions, Tragedy of the Commons, Ecosystem Services, Eutrophication, Human Impact on Aquatic Ecosystems, Aquaculture This case study explores the unique life cycle of oysters (Family Ostreidae) and their impact on aquatic marine environment, biogeochemical cycles, humans, and the economy. both on the food web and the nitrogen cycle. Answer key included.

Case Study: Oysters (Teacher & Student Edition)
File format.
ZIP file containing 2 PDF files (1 Teacher Edition and 1 Student Edition)
Curriculum Alignment
Next Generation Science Standards (NGSS): HS-LS2-1. Use mathematical and/or computational representations to support explanations of factors that affect carrying capacity of ecosystems at different scales. HS-LS2-2. Use mathematical representations to support and revise explanations based on evidence about factors affecting biodiversity and populations in ecosystems of different scales. HS-LS2-6. Evaluate the claims, evidence, and reasoning that the complex interactions in ecosystems maintain relatively consistent numbers and types of organisms in stable conditions, but changing conditions may result in a new ecosystem. HS-LS2-7. Design, evaluate, and refine a solution for reducing the impacts of human activities on the environment and biodiversity. HS-ESS2-5. Plan and conduct an investigation of the properties of water and its effects on Earth materials and surface processes. HS-ESS2-6. Develop a quantitative model to describe the cycling of carbon among the hydrosphere, atmosphere, geosphere, and biosphere. HS-ESS3-4. Evaluate or refine a technological solution that reduces impacts of human activities on natural systems.
AP® Environmental Science Learning Objectives: 1.5 Explain the steps and reservoir interactions in the nitrogen cycle. 1.7 Explain the steps and reservoir interactions in the hydrologic cycle. 1.9 Explain how energy flows and matter cycles through trophic levels. 1.10 Determine how the energy decreases as it flows through ecosystems. 1.11 Describe food chains and food webs, and their constituent members by trophic level. 2.3 Describe ecological tolerance. Describe the role of island biogeography in evolution. 2.4 Describe ecological tolerance. 3.4 Describe carrying capacity. Describe the impact of carrying capacity on ecosystems. 3.5 Explain how resource availability affects population growth. 5.1 Explain the concept of the tragedy of the commons. 5.8 Describe causes of and problems related to overfishing. 5.16 Describe the benefits and drawbacks of aquaculture. 8.2 Describe the impacts of human activities on aquatic ecosystems. 8.5 Explain the environmental effects of excessive use of fertilizers and detergents on aquatic ecosystems.
AP® Environmental Science Practices: 1: Explain environmental concepts, processes, and models presented in written format. 2: Analyze visual representations of environmental concepts and processes. 3: Analyze sources of information about environmental issues. 4: Analyze research studies that test environmental principles. 5: Analyze and interpret quantitative data represented in tables, charts, and graphs. 6: Apply quantitative methods to address environmental concepts. 7: Propose and justify solutions to environmental problems.
AP® is a registered trademark registered by the College Board®. This work/product/service has been developed independently from and is not endorsed by the College Board®.
- Research article
- Open access
- Published: 12 October 2011
Complete mitochondrial DNA sequence of the European flat oyster Ostrea edulis confirms Ostreidae classification
- Gwenaelle Danic-Tchaleu 1 ,
- Serge Heurtebise 1 ,
- Benjamin Morga 1 &
- Sylvie Lapègue 1
BMC Research Notes volume 4 , Article number: 400 ( 2011 ) Cite this article
4914 Accesses
22 Citations
Metrics details
Because of its typical architecture, inheritance and small size, mitochondrial (mt) DNA is widely used for phylogenetic studies. Gene order is generally conserved in most taxa although some groups show considerable variation. This is particularly true in the phylum Mollusca, especially in the Bivalvia. During the last few years, there have been significant increases in the number of complete mitochondrial sequences available. For bivalves, 35 complete mitochondrial genomes are now available in GenBank, a number that has more than doubled in the last three years, representing 6 families and 23 genera. In the current study, we determined the complete mtDNA sequence of O. edulis , the European flat oyster. We present an analysis of features of its gene content and genome organization in comparison with other Ostrea , Saccostrea and Crassostrea species.
The Ostrea edulis mt genome is 16 320 bp in length and codes for 37 genes (12 protein-coding genes, 2 rRNAs and 23 tRNAs) on the same strand. As in other Ostreidae, O. edulis mt genome contains a split of the rrnL gene and a duplication of trnM . The tRNA gene set of O. edulis , Ostrea denselamellosa and Crassostrea virginica are identical in having 23 tRNA genes, in contrast to Asian oysters, which have 25 tRNA genes (except for C. ariakensis with 24). O. edulis and O. denselamellosa share the same gene order, but differ from other Ostreidae and are closer to Crassostrea than to Saccostrea . Phylogenetic analyses reinforce the taxonomic classification of the 3 families Ostreidae, Mytilidae and Pectinidae. Within the Ostreidae family the results also reveal a closer relationship between Ostrea and Saccostrea than between Ostrea and Crassostrea .
Conclusions
Ostrea edulis mitogenomic analyses show a high level of conservation within the genus Ostrea , whereas they show a high level of variation within the Ostreidae family. These features provide useful information for further evolutionary analysis of oyster mitogenomes.
Because of its typical architecture, inheritance and small size, animal mitochondrial (mt) DNA is widely used for phylogenetic studies. Combined with these characteristics, its typically maternal inheritance contributes to a fast rate of evolution. Nucleotide changes combined with gene order and rearrangement data can provide valuable information on major evolutionary changes at different taxonomic levels. Typically, animal mtDNA is a compact molecule (14 to 17 kb), though some mtDNA can be vastly larger (e.g., Plactopecten magellanicus [ 1 ]), and usually encodes 13 proteins, 22 transfer RNAs (tRNAs) and 2 ribosomal RNAs (rRNAs) [ 2 ]. There are often few intergenic nucleotides except for a single large non-coding region generally thought to contain elements that control the initiation of replication and transcription [ 3 ]. Size variation in mtDNA is usually due to the different length of the non-coding regions. Gene order is generally conserved in most taxa, although some groups show considerable variation. This is particularly so in the Mollusca phylum, especially in Bivalvia and Scaphopoda [ 4 ]. In addition to the fact that phylogenetic relationships among major molluscan groups are not well understood, the species classification of some of the most common mollusks remains difficult.
A case in point is oysters, for which a plastic growth pattern is a major feature, resulting in a wide range of overlapping ecophenotypic variants [ 5 , 6 ]. Oysters are bivalve molluscs that are widely distributed in the world's oceans. As benthic, sessile filter-feeders, they play an important role in estuarine ecosystems. Moreover, some species are of economic importance, like the Pacific cupped oyster, which is grown in 27 countries and is the most highly produced mollusc species in the world. Oysters have been introduced all over the world for culture and many species are sympatric. Numerous species (30-40 according to the classifications) of oysters of the genus Ostrea have been described. Their geographical range is particularly wide in warm and temperate waters of all oceans, although they have a predominantly tropical distribution [ 6 , 7 ]. In Europe, along the Atlantic and Mediterranean coasts, the European flat oyster, Ostrea edulis , is an important economic marine resource: in 2009 almost 3000 tons were produced in the world, mainly (91%) in Europe (Spain, France, Ireland ...) [ 8 ].
During the last few years, there have been significant increases in the number of complete mitochondrial sequences available for all species. The number has more than doubled for molluscs in the last three years [ 9 ], so that 98 complete mollusk mitochondrial genomes are now available in GenBank, mainly from gastropods (43), bivalves (35) and cephalopods (14). Among bivalves, the sequenced genomes represent 6 families and 23 genera. In the Ostreidae, the genus Crassostrea has been thoroughly studied, with 7 representatives (6 Asian oysters and 1 American oyster) [ 10 ]. In contrast, there is only one representative of the genus Saccostrea ( Saccostrea mordax ), and one of the genus Ostrea ( Ostrea denselamellosa ). Recent studies have provided a more comprehensive picture of the cupped oyster genome, showing an unusually high conservation of mitochondrial gene order in Asian Crassostrea species [ 11 ]. Even though molecular tools, such as mitochondrial or microsatellite markers, already exist for the European flat oyster and allow population genetics [ 12 ] or quantitative genetics [ 13 ] studies, the complete characterization of its mtDNA will allow a better study to be made of phylogenetic relationships among members of the genus, especially between the closely-related species O. edulis and O. angasi [ 14 ], to improve classification of the Ostreidae family within the Bivalvia.
Results and discussion
Genome composition.
The complete mitochondrial genome of Ostrea edulis [GenBank: JF274008] is 16 320 nt in length and encodes 37 genes, including 12 protein-coding genes (PCGs), 2 rRNAs and 23 tRNAs on the same strand (Figure 1 and Table 1 ). This size is very close to that of O. denselamellosa (16 277 bp), shorter than that of other Ostreidae (16 532 bp for S. mordax to 22 446 bp for C. iredalei ), and is within the size range of the Pteriomorphia mt genomes published to date: from 16 211 nt for Argopecten irradians [ 15 ] to 32 115 nt for Placopecten magellanicus [ 1 ].
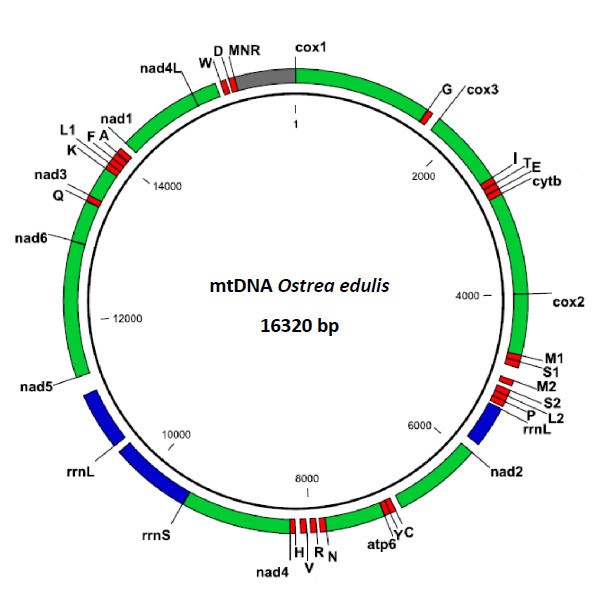
Mitochondrial genome map of Ostrea edulis . Genes for proteins (green) and rRNAs (blue) are abbreviated with standard abbreviations. The one letter amino acid code is used for tRNA (red) designation. The major non-coding region (MNR) is shown in grey.
In the mt genome of O. edulis , a total of 965 bp of non-coding nucleotides is spread over 21 intergenic regions (each over 5 bp) including a major non-coding region (MNR) of 695 bp. A large non-coding region suggests a putative control region based on its AT content of 74.4% [ 16 ]. In contrast to typical animal mitochondrial genomes, the O. edulis genome may lack the protein-coding gene atp8 , although some recent studies have found a good candidate for atp8 gene in Mytilidae and possibly in some Ostreidae [ 17 ]. Furthermore, O. edulis genome also has duplications of three tRNAs: trnM , trnS and trnL . The rrnL gene is split into 2 fragments, a phenomenon previously observed in the Ostreidae [ 11 ]. The rrnS is not duplicated (also in O. denselamellosa , S. mordax and C. virginica ), in contrast to Asian Crassostrea .
The molecule has an overall A+T composition value of 64.9% and the size of the coding region is 15 379 nt in length, accounting for 94.2% of the whole genome. The AT content is slightly higher than those of Pectinidae (55.3 to 59.6% [ 18 ]) or Mytilidae (61.5 to 61.8%). The AT composition of O. edulis is, therefore, within the AT content range of the Ostreidae: the lowest known AT content is 60.7% in O. denselamellosa , while the highest is 65.3% in C. hongkongensis [ 19 ]. In S. mordax , the AT content is 64.4% which is very similar to O. edulis .
As observed in O. denselamellosa (16 277 bp), S. mordax (16,532 bp) and C. virginica (17 244 bp), the lack of duplicated rrnS in O. edulis , added to the lack of 2 tRNAs (not duplicated trn-K and trn-Q ) compared to Asian Crassostrea may account for the difference in length compared with other Crassostrea ( C. gigas 18225 bp, C. hongkongensis 18 622 bp).
The genome composition of O. edulis is, thus, identical to O. denselamellosa (except for AT composition) and close to S. mordax in terms of complete genome size, AT content and the non-duplicated rrnS . More mitochondrial genome sequences from Ostrea and Saccostrea will be needed to assess relationships between the Ostrea , Crassostrea and Saccostrea genera .
Gene arrangement
Animal mt gene order is relatively stable within major groups and generally variable among groups [ 2 ]. Bivalve species show variability in terms of mt genome size, gene arrangement and tRNA number [ 20 ]. As observed in four Pectinidae ( A. irradians , M. yessoensis , C. farreri and P. magellanicus ), gene arrangement can be very different despite species being members of the same family [ 15 ]. In contrast, in Mytilus congeners M. edulis , M. trossulus and M. galloprovincialis , PCGs are arranged in an identical order, but tRNA, rRNA and control regions are also almost the same [ 21 ]. The mt gene order of O. edulis is identical to that of O. denselamellosa (Figure 2 ). The arrangement of PCGs in O. edulis is cox1 , cox3 , cytb , cox2 , nad2 , atp6 , nad4 , nad5 , nad6 , nad3 , nad1, nad4L and is nearly identical to that of C rassostrea , except for the inversion of two PCGs nad2 and atp6 . Among the six Asian Crassostrea and their Atlantic sister species C. virginica , only protein-coding gene order is identical [ 10 ]. Besides, gene order of Crassostrea differs significantly from S. mordax . Ostreidae share three PCG blocks cox1-cox3-cytb-cox2 , nad5-nad6 and nad3-nad1-nad4L . Moreover, the nad2-atp6-nad4 block of O. edulis is inverted in S. mordax (Figure 2 ), but the remaining genes are extensively rearranged. The major non-coding region ( MNR) is located after trnD (Figure 1 ), while this region is found between trnG and trnV near atp6 and nad2 in Crassostrea . If tRNAs and rRNAs are considered, there are six blocks conserved within the Ostreidae: cox3-trnI, cox2-trnM, trnM-trnS, trnY-atp6, trnH-nad4 and trnF-trnA-nad1-nad4L . Between O. edulis and Asian Crassostrea , seven blocks are shared: cox3-trnI-trnT-trnE-cytb , cox2-trnM-trnS, trnM-trnS, trnY-atp6, trnH-nad4, nad5-nad6-trnQ-nad3 and trnL-trnF-trnA-nad1-nad4L-trnW ; while between O. edulis and S. mordax , seven blocks are also shared but these are different: trnG-cox3-trnI, trnE-cytb-cox2-trnM, trnM-trnS-trnL-trnP-rrnL(5'end) , trnF-trnA-nad1-nad4L, rrnL(3'end)-nad5-nad6, trnH-nad4 and trnY-atp6 . It should be noted that rrnL is in one piece in Saccostrea but not within Crassostrea .
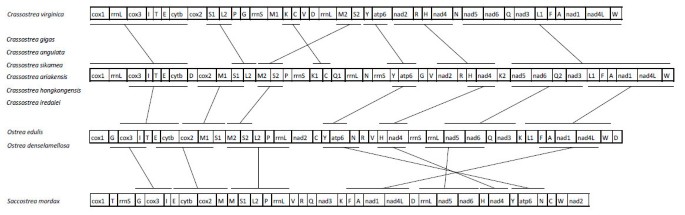
The gene arrangement map of Ostreidae mitochondrial genomes . The bars show identical gene blocks. All genes are transcribed from left-to-right.
In terms of gene arrangement, it is thus clear that O. edulis is more similar to Crassostrea than to S. mordax when comparing PCGs. As shown in Figure 2 , the complete genome arrangement of O. edulis is similar to that of Asian Crassostrea while it appears completely reorganized from trnY to the end of mt genome when compared with that of S. mordax .
Protein-coding genes
All PCGs are encoded on and transcribed from the same strand. Twelve open reading frames (ORFs) were detected for the thirteen typical PCGs ( cox1-cox3, cytb, nad1-nad6, nad4L, atp6 and atp8 ). Although we carefully looked for candidate regions for atp8 gene, we could not identify any, as in all Pteriomorphia complete genomes already published. However, a recent publication [ 17 ] suggests that a putative ORF represents a good candidate to start an atp8 gene in most bivalve mt genomes. Within the invertebrate mt code there are three standard initiation codons (M-AUG, M-AUA, and I-AUU), but mt genomes often use a variety of non conventional start codons [ 22 ]. In this study, most of PCGs use conventional initiation codons: ATA is used for cox3, nad4, nad6 and atp6 ; ATG is used for cox1, cox2, nad1, nad3 , nad4L and nad5 ; ATT is used for nad2 , but cytb uses the alternative start codon CTA (as in C. gigas and C. angulata [ 10 ]). Eight protein-coding genes were terminated by a stop codon (TAA and TAG).
Transfer and ribosomal RNA genes
In total, 23 tRNA coding genes were identified in the size range of 63 to 71 nucleotides, based on typical secondary structure (Additional file 1 ). An additional trnM was detected as found in C. gigas , C. hongkongensis [ 9 ], C. virginica [ 16 ] and Mytilus [ 23 ]. Two serine and two leucine tRNA genes were also differentiated in O. edulis by their anticodons (UCA Ser1, AGA Ser2, and CUA Leu1, UUA Leu2) as found in O. denselamellosa [ 24 ], Crassostrea and some other species ( M. edulis , M. galloprovincialis and Argopecten irradians ). The anticodon usage of O. edulis was congruent with the corresponding tRNA genes of other molluscan mtDNAs.
Identification of both the small and the large ribosomal RNA genes in O. edulis was accomplished by BLAST comparison with other published ribosomal RNA genes, especially O. denselamellosa [GenBank:HM015199], S. mordax [GenBank:FJ841968] and C. gigas [GenBank:EU672831]. Although putative gene boundaries for the two rRNA genes have been found, these cannot be precisely determined until transcript mapping is carried out. Besides rrnS of O. edulis is 935 bp in length and flanked by nad4 and rrnL 3'end .
The rrnL gene is split into two segments: one segment, of the 5' end (matches with rrnL 5'end from O. denselamellosa and Saccostrea ), is 575 bp long and positioned between trnP and nad2 ; and the other segment, of the 3' end, is 708 bp and located between rrnS and nad5 . The length of the rrnS is similar to that of most bivalves, but smaller than that of O. denselamellosa (1017 bp) and that of Crassostrea (946 to 1207 bp) [ 10 ]. The size of rrnL (1283 bp in all) is similar to that of O. denselamellosa (1299 bp), but smaller than that of other bivalves. This bias may be due to the method (BLAST) used to compare the rRNA sequences because this method only checks the identity between a few sequences and because it's easier to compare sequences from same species as they show higher identity.
Non-coding regions
As in most bivalves, O. edulis mtDNA contains a large number of unassigned nucleotides. There are as many as 21 non-coding regions (> 5 bp) up to 965 nucleotides found throughout the O. edulis mitochondrial genome. Eight of these non-coding regions are more than 50 bp in length. Among these regions, the major non-coding region (MNR) has been identified and located, that remains the most promising region in which to find regulatory and/or gender-specific sequences [ 25 ]. The O. edulis mtDNA MNR is positioned between trnD and cox1 and is 695 bp in length, similar to that of O. denselamellosa (689 bp), making it the longest MNR within the Ostreidae apart from C. virginica (832 bp) and C. ariakensis (716 bp). It has an A+T content of 74.4% which is higher than the remainder of the mt genome (64.4%), as it includes several (A)n and (T)n homopolymer tracts, features which are typically used for identification of the mitochondrial control region and thought to contain the replication origin [ 2 ].
Phylogenetic analysis
In recent years there have been many phylogenetic studies on the taxonomy and evolution of the Ostreidae based on molecular data, especially mitochondrial DNA [ 26 – 30 ]. However, most of these previous studies have been based on partial sequences and incomplete molecular information. Recently, Ren et al. [ 11 ] have compared 7 complete mt genomes from Asian oysters.
In the present study's aa-based tree built with twelve concatened PCGs from 19 mitochondrial genomes in Pteromorphia (Figure 3 ), we can observe that, at the Ostreidae level, O. edulis is first clustered with O. denselamellosa as congeneric species. Then this group of species falls into a highly supported clade with S. mordax. Ostrea and Saccostrea are then clustered with the Crassostrea species group. In this latest clade, the single American oyster C. virginica falls at the base of a nested clade that contains the Asian oysters. Very similar results were obtained with a nucleotide phylogenetic tree with low differences of bootstrap values. In Figure 4 , more Ostreidae species are included as more numerous cox1 sequences are available in Genbank. The same phylogenetic relationship between Ostrea , Saccostrea , and Crassostrea is observed, especially the first grouping of Ostrea and Saccostrea , but not between Ostrea and Crassostrea , with however far less robust nodes. This same result was observed when considering the evolution of the tRNA anticodons in marine bivalve mitochondrial genomes, where the relationship presented are also based on concatenated nucleotide sequences of 12 protein-coding genes by Bayesian inference analysis [ 24 ]. However a recent study [ 31 ], based on cox1 and 16S sequences, showed a closer relationship between Ostrea and Crassostrea , than with Ostrea and Saccostrea . However, for the cox1 analysis, only one Ostrea sequence was included, and for the 16S analysis, much more Ostrea sequences were included but the bootstrap value was between 50 and 80%. Those comparisons seem to indicate that phylogenetic analyses are more powerful when including several sequences as the 12 concatened PCGs.
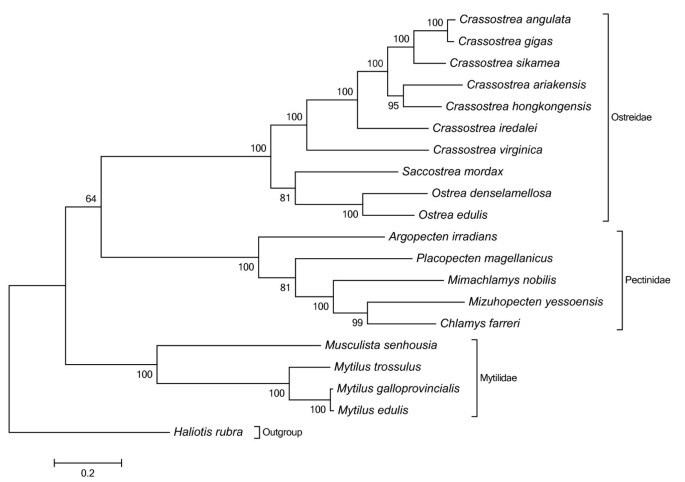
Phylogenetic tree based on twelve concatenated PCGs from 19 mitochondrial genomes .
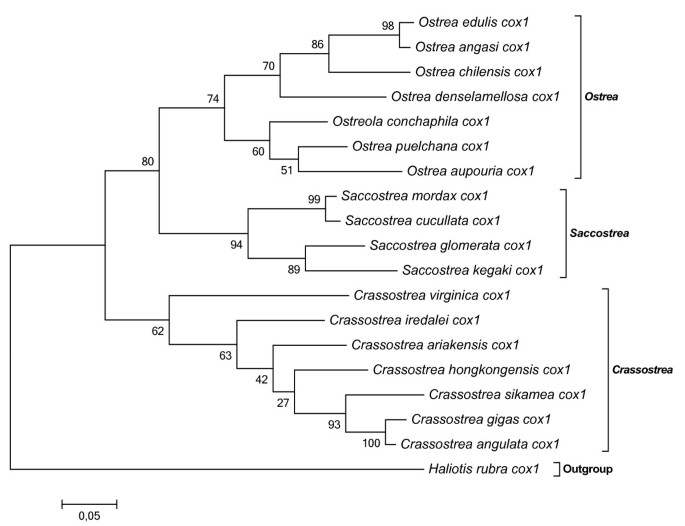
Phylogenetic tree based on cox1 from all published Ostrea , Ostreola , Saccostrea and Crassostrea .
Finally, the phylogenetic tree presented in Figure 3 , which includes mt genomes from all published Pteriomorphia, reinforces the taxonomic classification of the 3 families Ostreidae, Mytilidae and Pectinidae [ 32 , 11 ].
In conclusion, the complete mitochondrial genome of O. edulis is 16 320 bp in length. A common phenomenon is that mitogenomes of most bivalves contain two trnM genes and most metazoan mitochondria have a set of 22 tRNA, including two trnL and two trnS . However the tRNA gene sets of O. edulis , O. denselamellosa and C. virginica are identical in having 23 tRNA genes. Another important characteristic is that the rrnS gene is not duplicated in O. edulis , a feature shared with O. denselamellosa , S. mordax and C. virginica and which contrasts with Asian Crassostrea .
The phylogenetic analyses confirm the relationships between each family (Ostreidae, Mytilidae and Pectinidae), but also within each genus ( Ostrea , Saccostrea and Crassostrea ). Within the Ostreidae, phylogenetic analyses show that Ostrea are closer to Saccostrea than Crassostrea , although gene arrangement may show a closer relationship between Ostrea and Crassostrea , indicating that several types of information are needed to infer relationships between genome species as evolution is acting at different levels of the genomes. As many questions remain unanswered on the phylogeny of Ostreidae, especially between Ostrea and Saccostrea , it would be desirable to increase the resolution by adding samples of more taxa in order to extend molecular information among the major lineages of the Ostreidae and within the Pteriomorphia as a whole.
PCR amplification and DNA sequencing
Adductor muscle from three O. edulis collected in Quiberon Bay (Bretagne, France) was used in this study. Total genomic DNA was extracted using a Wizard ® DNA Clean-up System (Promega). The mitochondrial genome was amplified in 4 overlapping fragments using species-specific primers (Additional file 2 ). PCR was performed in 25 μl reaction volumes in a thermocycler (Applied Biosystems). Each reaction contained 13.3 μl dH2O, 5.0 μl buffer 5× (Promega), 2.0 μl MgCl2 (25 mM), 2.5 μl dNTP (2 mM), 0.5 μl of each primer (20 μM), and 0.2 μl GoTaq ® DNA polymerase (5U/μl, Promega). PCR cycling conditions were 94 °C for 2 min; then 30 cycles of 94 °C for 30 sec, 57 °C for 30 sec and 72 °C for 2 min; and finally a step of 72 °C for 10 min. PCR products were verified by electrophoresis (1% agarose gel) and purified using Montage ® PCR Centrifugal Filter Devices (Millipore). Purified products were then used directly as templates in cycle sequencing reactions with dyelabeled terminators (Big Dye 3.1, Applied Biosystems). Specific primers were designed and used for primer walking sequencing, which was performed for both strands of each sample on an ABI 3130XL/Genetic Analyser (ABI).
Sequence analysis and gene annotation
During the processing of large fragments and those from primer walking sequencing, regular and manual examinations were used to ensure there was reliable overlapping and correct genome assembly.
Protein-coding and ribosomal RNA genes were firstly identified using BLAST [ 33 ] searches at GenBank, and then by alignment with previously published mt genomes from species of Crassostrea , Saccostrea and other closely-related molluscs. Amino-acid sequences of protein-coding genes were inferred with ORF Finder [ 34 ] using invertebrate mitochondrial genetic code. Transfer RNAs were identified using DOGMA [ 35 ] http://dogma.ccbb.utexas.edu/ , and tRNAscan-SE [ 36 ] http://selab.janelia.org/tRNAscan-SE/ using mito/chloroplast genetic code and default search mode, or setting the cove cutoff score to 1 when necessary. Assembly of the genome and gene map of the mitochondrial genome of Ostrea edulis was performed using CLC Main Workbench (CLC bio).
To date, 20 Pteriomorphia mt genomes are available in GenBank [ 37 ] and we used 19 of these (excluding Argopecten irradians irradians that is very close to Argopecten irradians : 99% similarity) in our phylogenetic analysis, together with O. edulis mt genome obtained in this study (Table 2 ). The blacklip abalone Haliotis rubra (Gastropoda) was used as the outgroup. The nucleotide and amino-acid sequences from all 12 PCGs (protein-coding genes) were concatenated for each genome and aligned using MUSCLE [ 38 ]. The size of the concatenated alignment nucleotides varied from 10 411 bp for M. yessoensis to 11 240 bp for P. magellanicus ). To the alignments, we applied a Maximum Likelihood (ML) phylogenetic reconstruction approach using 100 bootstraps with MEGA5 [ 39 ]. A second phylogenetic analysis was performed using 5 additional cox1 genes from Ostrea ( Ostrea angasi [GenBank:AF112287.1], Ostrea aupouria [GenBank:AF112288.1], Ostrea chilensis [GenBank:AF112289.1], Ostrea puelchana [GenBank:DQ226521.1], and Ostreola conchaphila [GenBank:DQ464125.1]) and 3 from Saccostrea ( Saccostrea cucullata [GenBank:AY038076.1], Saccostrea glomerata [GenBank:EU007483.1] and Saccostrea kegaki [GenBank:AB076910.1]).
Smith DR, Snyder M: Complete Mitochondrial DNA sequence of the scallop Placopecten magellanicus : evidence of transposition leading to an uncharacteristically large mitochondrial genome. J Mol Evol. 2007, 65: 380-391. 10.1007/s00239-007-9016-x.
Article PubMed CAS Google Scholar
Boore JL: Animal mitochondrial genomes. Nucleic Acids Res. 1999, 27: 1767-1780. 10.1093/nar/27.8.1767.
Article PubMed CAS PubMed Central Google Scholar
Shadel GS, Clayton DA: Mitochondrial DNA maintenance in vertebrates. Annu Rev Biochem. 1997, 66: 409-435. 10.1146/annurev.biochem.66.1.409.
Serb JM, Lydeard C: Complete mtDNA sequence of the North American freshwater mussel, Lampsilis ornate (Unionidae): An examination of the evolution and phylogenetic utility of mitochondrial genome organization in Bivalvia (Mollusca). Mol Biol Evol. 2003, 20: 1854-1866. 10.1093/molbev/msg218.
Ranson G: Les huîtres: biologie, culture. 1951, Paul Lechevalier, Paris
Google Scholar
Barnabe G: Aquaculture: biology and ecology of cultured species. 1994, Ellis Horwood Series in Aquaculture and Fisheries Support, Wiley & Sons, Chichester, UK
Book Google Scholar
Jaziri H: Variations génétiques et structuration biogéographique chez un bivalve marin: l'huître plate Ostrea edulis (L). PhD thesis. 1990, Université Montpellier II--Sciences et Techniques du Languedoc
FAO: Global Aquaculture production 1950-2009. 2009, [ http://www.fao.org/fishery/statistics/global-aquaculture-production/en ]
Yu Z, Wei Z, Kong X, Shi W: Complete mitochondrial DNA sequence of oyster Crassostrea hongkongensis -a case of "Tandem duplication-random loss" for genome rearrangement in Crassostrea ?. BMC Genomics. 2008, 9: 477-10.1186/1471-2164-9-477.
Article PubMed PubMed Central Google Scholar
Wu X, Xu X, Yu Z, Wei Z, Xia J: Comparison of seven Crassostrea mitogenomes and phylogenetic analyses. Molecular Phylogenetics and Evolution. 2010, 57 (1): 448-454. 10.1016/j.ympev.2010.05.029.
Ren J, Liu X, Jiang F, Guo X, Liu B: Unusual conservation of mitochondrial gene order in Crassostrea oysters: evidence for recent speciation in Asia. BMC Evolutionary Biology. 2010, 10: 394-10.1186/1471-2148-10-394.
Diaz-Almela E, Boudry P, Launey S, Bonhomme F, Lapègue S: Comparison of mitochondrial DNA and microsatellites variation along the range of the European flat oyster Ostrea edulis . J Hered. 2004, 95: 510-516. 10.1093/jhered/esh073.
Lallias D, Stockdale R, Boudry P, Beaumont AR, Lapègue S: Characterization of 27 microsatellite loci in the European flat oyster Ostrea edulis . Molecular Ecology Resources. 2009, 9 (3): 960-963. 10.1111/j.1755-0998.2009.02515.x.
Morton B, Lam K, Black-Smith S: First report of the European flat oyster Ostrea edulis , identified genetically, from oyster harbour, Albany, South-Western Australia. Molluscan Research. 2003, 23: 199-208. 10.1071/MR03005.
Article Google Scholar
Ren J, Shen X, Jiang F, Liu B: The mitochondrial genomes of two scallops, Argopecten irradians and Chlamys farreri (Mollusca: Bivalvia): the most highly rearranged gene order in the family Pectinidae. J Mol Evol. 2010, 70 (1): 57-68. 10.1007/s00239-009-9308-4.
Milbury CA, Gaffney PM: Complete mitochondrial DNA sequence of the eastern oyster Crassostrea virginica . Mar Biotechnol. 2005, 7: 697-712. 10.1007/s10126-005-0004-0.
Breton S, Stewart DT, Hoeh WR: Characterization of a mitochondrial ORF from the gender-associated mtDNAs of Mytilus spp. (Bivalvia: Mytilidae): Identification of the "missing" ATPase 8 gene. Marine Genomics. 2010, 3: 11-18. 10.1016/j.margen.2010.01.001.
Article PubMed Google Scholar
Wu X, Xu X, Yu Z, Kong X: Comparative mitogenomic analyses of three scallops (Bivalvia: Pectinidae) reveal high level variation of genomic organization and a diversity of transfer RNA gene sets. BMC Res Notes. 2009, 2: 69-10.1186/1756-0500-2-69.
Ren J, Liu X, Zhang G, Liu B, Guo X: ''Tandem duplication-random loss" is not a real feature of oyster mitochondrial genomes. BMC Genomics. 2009, 10: 84-10.1186/1471-2164-10-84.
Gissi C, Iannelli F, Pesole G: Evolution of the mitochondrial genome of Metazoa as exemplified by comparison of congeneric species. Heredity. 2008, 101: 301-10.1038/hdy.2008.62.
Mizi A, Zouros E, Moschonas N, Rodakis GC: The complete maternal and paternal mitochondrial genomes of the Mediterranean mussel Mytilus galloprovincialis : implications for the doubly uniparental inheritances mode of mtDNA. Mol Biol Evol. 2005, 22: 952-967. 10.1093/molbev/msi079.
Wolstenholme DR: Animal mitochondrial DNA: structure and evolution. Int Rev Cytol. 1992, 141: 173-216.
Hoffman RJ, Boore JL, Brown WM: A novel mitochondrial genome organization for the blue mussel, Mytilus edulis . Genetics. 1992, 131: 397-412.
Yu H, Li Q: Mutation and Selection on the Wobble Nucleotide in tRNA Anticodons in Marine Bivalve Mitochondrial Genomes. PLoS One. 2011, 186 (1): e16147-
Breton S, Doucet Beaupré H, Stewart DT, Piontkivsha H, Karmakar M, Bogan AE, Blier PU, Hoeh WR: Comparative mitochondrial genomics of freshwater mussels (Bivalvia: Unionoida) with Doubly Uniparental Inheritance of mtDNA: gender-specific Open Reading Frames (ORFs) and putative origins of replication. Genetics. 2009, 183: 1575-1589. 10.1534/genetics.109.110700.
Boore JL, Brown WM: Big trees from little genomes: mitochondrial gene order as a phylogenetic tool. Curr Opin Genet Dev. 1998, 8: 668-674. 10.1016/S0959-437X(98)80035-X.
Boudry P, Heurtebise S, Collet B, Cornette F, Gérard A: Differentiation between populations of the Portuguese oyster, Crassostrea angulata (Lamarck), and the Pacific oyster, Crassostrea gigas (Thunberg), revealed by mtDNA RFLP analysis. Journal of Experimental Marine Biology and Ecology. 1998, 226: 279-291. 10.1016/S0022-0981(97)00250-5.
Klinbunga S, Khamnamtong B, Puanglarp N, Jarayabhand P, Yoosukh W, Menasveta P: Molecular taxonomy of cupped Oysters ( Crassostrea , Saccostrea and Striostrea ) in Thailand based on COI, 16S, and 18S rDNA polymorphism. Marine Biotechnology. 2005, 7: 306-317. 10.1007/s10126-004-0036-x.
Lam K, Morton B: Morphological and mitochondrial-DNA analysis of the Indo-West Pacific rock oysters (Ostreidae Saccostrea species). J Mollus Stud. 2006, 72 (3): 235-245. 10.1093/mollus/eyl002.
Reece KS, Cordes JF, Stubbs JB, Francis E, Hudson K: Molecular phylogenies help resolve taxonomic confusion with Asian Crassostrea oyster species. Marine Biology. 2008, 153: 709-721. 10.1007/s00227-007-0846-2.
Liu J, Li Q, Kong L, Yu H, Zheng X: Identifying the true oysters (Bivalvia: Ostreidae) with mitochondrial phylogeny and distance-based DNAbarcoding. Molecular Ecology Resources. 2011, 11: 820-830. 10.1111/j.1755-0998.2011.03025.x.
Giribet G, Wheeler W: On bivalve phylogeny: a high-level analysis of the Bivalvia (Mollusca) based on combined morphology and DNA sequence data. Invertebrate Biology. 2002, 121 (4): 271-324.
The Basic Local Alignment Search Tool (BLAST). [ http://www.ncbi.nlm.nih.gov/BLAST/ ]
NCBI Open Reading Frame Finder. [ http://www.ncbi.nlm.nih.gov/gorf/gorf.html ]
Wyman SK, Jansen RK, Boore JL: Automatic annotation of organellar genomes with DOGMA. Bioinformatics. 2004, 20 (17): 3252-3255. 10.1093/bioinformatics/bth352. [ http://dogma.ccbb.utexas.edu/ ]
Lowe TM, Eddy SR: A program for improved detection of transfer RNA genes in genomic sequence. tRNAscan-SE Search Server. Nucl Acids Res. 1997, 25: 955-964. 10.1093/nar/25.5.955. [ http://selab.janelia.org/tRNAscan-SE/ ]
GenBank. [ http://www.ncbi.nlm.nih.gov/ ]
Edgar RC: MUSCLE: multiple sequence alignment with high accuracy and high throughput. Nucl Acids Res. 2004, 32 (5): 1792-1797. 10.1093/nar/gkh340.
Tamura K, Peterson D, Peterson N, Stecher G, Nei M, Kumar S: MEGA5: Molecular Evolutionary Genetics Analysis using Maximum Likelihood, Evolutionary Distance, and Maximum Parsimony Methods. Molecular Biology and Evolution. 2011, 28 (10): 2731-2739. 10.1093/molbev/msr121.
Download references
Acknowledgements
The authors thank Aimé Langlade who provided biological material, Denis Saulnier who gave valuable advice, Nicole Faury for her technical assistance and Helen McCombie for English editing. We also thank two anonymous reviewers for their useful comments on the manuscript.
Author information
Authors and affiliations.
IFREMER, Laboratoire de Génétique et Pathologie, F-17390, La Tremblade, France
Gwenaelle Danic-Tchaleu, Serge Heurtebise, Benjamin Morga & Sylvie Lapègue
You can also search for this author in PubMed Google Scholar
Corresponding author
Correspondence to Sylvie Lapègue .
Additional information
Competing interests.
The authors declare that they have no competing interests.
Authors' contributions
GDT, SH and BM obtained the sequences. GDT and SL performed analyses and wrote the first draft of the publication. All the co-authors finalised the manuscript.
Electronic supplementary material
13104_2011_1183_moesm1_esm.pdf.
Additional file 1:The potential secondary structures of 22 tRNAs of Ostrea edulis . The duplication of methionine is named M1 and M2 respectively. Codons recognized are shown for the pairs of leucine (L1 and L2) and serine (S1 and S2). (PDF 115 KB)
13104_2011_1183_MOESM2_ESM.PDF
Additional file 2:Primers used for amplification of 4 large fragments in mitochondrial genome of Ostrea edulis .(PDF 223 KB)
Authors’ original submitted files for images
Below are the links to the authors’ original submitted files for images.
Authors’ original file for figure 1
Authors’ original file for figure 2, authors’ original file for figure 3, authors’ original file for figure 4, rights and permissions.
This article is published under license to BioMed Central Ltd. This is an open access article distributed under the terms of the Creative Commons Attribution License ( http://creativecommons.org/licenses/by/2.0 ), which permits unrestricted use, distribution, and reproduction in any medium, provided the original work is properly cited.
Reprints and permissions
About this article
Cite this article.
Danic-Tchaleu, G., Heurtebise, S., Morga, B. et al. Complete mitochondrial DNA sequence of the European flat oyster Ostrea edulis confirms Ostreidae classification. BMC Res Notes 4 , 400 (2011). https://doi.org/10.1186/1756-0500-4-400
Download citation
Received : 09 June 2011
Accepted : 12 October 2011
Published : 12 October 2011
DOI : https://doi.org/10.1186/1756-0500-4-400
Share this article
Anyone you share the following link with will be able to read this content:
Sorry, a shareable link is not currently available for this article.
Provided by the Springer Nature SharedIt content-sharing initiative
- Mitochondrial Genome
- Complete Mitochondrial Genome
- Crassostrea Species
BMC Research Notes
ISSN: 1756-0500
- General enquiries: [email protected]
An official website of the United States government
Official websites use .gov A .gov website belongs to an official government organization in the United States.
Secure .gov websites use HTTPS A lock ( Lock Locked padlock icon ) or https:// means you've safely connected to the .gov website. Share sensitive information only on official, secure websites.
- Publications
- Account settings
- Advanced Search
- Journal List
Geometric morphometric methods for identification of oyster species based on morphology
Yuepeng guo, yanzhuo yang, haijiao liu, zhenlin hao.
- Author information
- Article notes
- Copyright and License information
Corresponding authors: Ying Tian ( [email protected] ), Zhenlin Hao ( [email protected] ).
Academic editor: Dimitris Poursanidis
Corresponding author.
Received 2023 Nov 6; Accepted 2024 Feb 3; Collection date 2024.
This is an open access article distributed under the terms of the Creative Commons Attribution License (CC BY 4.0), which permits unrestricted use, distribution, and reproduction in any medium, provided the original author and source are credited.
Both genetic and environmental factors affect the morphology of oysters. Molecular identification is currently the primary means of species identification, but it is inconvenient and costly. In this research, we evaluated the effectiveness of geometric morphometric (GM) techniques in distinguishing between two oyster species, Crassostrea gigas and C. ariakensis . We used traditional morphometric and GM methods, including principal component analysis (PCA), thin-plate spline analysis (TPS) and canonical variable analysis (CVA), to identify specific features that distinguish the two species. We found that differences in shape can be visualised using GM methods. The Procrustes analysis revealed significant differences in shell morphology between C. gigas and C. ariakensis . The shells of C. ariakensis are more prominent at the widest point and are more scattered and have a greater variety of shapes. The shells of C. gigas are more oval in shape. PCA results indicated that PC1 explained 45.22%, PC2 explained 22.09% and PC3 explained 10.98% of the variation between the two species, which suggests that the main morphological differences are concentrated in these three principal components. Combining the TPS analysis function plots showed that the shell shape of C. ariakensis is mainly elongated and spindle-shaped, whereas the shell shape of C. gigas is more oval. The CVA results showed that the classification rate for the two species reached 100% which means that C. ariakensis and C. gigas have distinct differences in shell morphology and can be completely separated, based on morphological characteristics. Through these methods, a more comprehensive understanding of the morphological characteristics of different oyster populations can be obtained, providing a reference for oyster classification and identification.
Keywords: traditional morphometrics, geometric morphometrics, Pacific oyster
Introduction
Oysters belong to the Phylum Mollusca , Class Bivalvia , Order Pterioida and Family Ostreidae ( Zhang and Lou 1956 , Ruppert 2013 , Bayne et al. 2017 ). Over 100 species of oysters have been discovered to date ( Lindberg and Ponder 2008 , Bayne et al. 2017 ). They have a worldwide distribution and are an important marine biological resource ( Bieler et al. 2010 , Wu et al. 2011 , Salvi et al. 2014 ). They are also important aquaculture species both domestically and internationally ( Dong et al. 2004 , Wijsman et al. 2018 , Bayne et al. 2019 ). In coastal areas of China, 30 species of oysters had been reported by Xu (1997) and the report in 2008 listed 23 species ( Xu and Zhang 2008 ). Recent research indicates that a total of 37 species of oysters have been discovered in China to date ( Li et al. 2017 , Guo et al. 2018 , Hu et al. 2019 , Cui et al. 2021 ).
Due to the susceptibility of oyster shells to environmental changes, oyster classification has always been controversial. The continuous study of oyster classification has resulted in a relatively mature oyster classification system ( Que et al. 2003 ). By sequencing and analysing the oyster genome, the classification position and relationship of oysters has become more accurate, providing new means for oyster classification research ( Wang et al. 2007 ). However, during practical production and aquaculture processes, the application of molecular techniques is not feasible for non-destructive classification of large numbers of specimens.
Morphometrics is a method for studying trait variation and its covariance with other variables ( Rohlf and Slice 1990 ). Currently, geometric morphometric (GM) methods are widely used in medicine ( Du and Lu 2006 ), botany ( Su et al. 2021 ) and biological classification ( Minton et al. 2008 , Perez 2011 , Miller 2016 ). Shu et al. (2022) used GM to analyse the morphological differences of eight scallop species in China. Jiang et al. (2019) successfully used GM to identify different geographic populations of the Chinese mitten crab ( Eriocheir sinensis ). Al-Kandari et al. (2021) stated that the current understanding of oyster evolutionary diversity is incomplete and that molecular data are crucial for oyster classification and identification. Molecular identification is currently the primary means of species identification, while it is inconvenient and costly. Comparing GM and molecular identification results is important for improving the accuracy of species classification, which would be highly valuable for oyster species classification.
The methods used in this study are traditional morphometric measurements, as well as multivariate linear analysis, principle component analysis (PCA), thin-plate spline analysis (TPS) and canonical variable analysis (CVA) to analyse the morphological differences between two oyster species ( Crassostrea gigas and C. ariakensis ).
Material and Methods
Sample collection.
In November 2022, oysters were randomly collected from two sites: Erjiegou ( 40.81°N, 121.97°E ) and Laohutan ( 38.90°N, 121.67°E ) in Liaoning Province, China (Fig. 1 ). A total of 57 (31 samples from Erjiegou and 26 samples from Laohutan) oysters were transported to the Key Laboratory of Mariculture and Stock Enhancement in the Northern Sea Area, Ministry of Agriculture and Rural Affairs, Dalian Ocean University for temporary culture. After removing surface attachments, the oysters were dissected and measured.
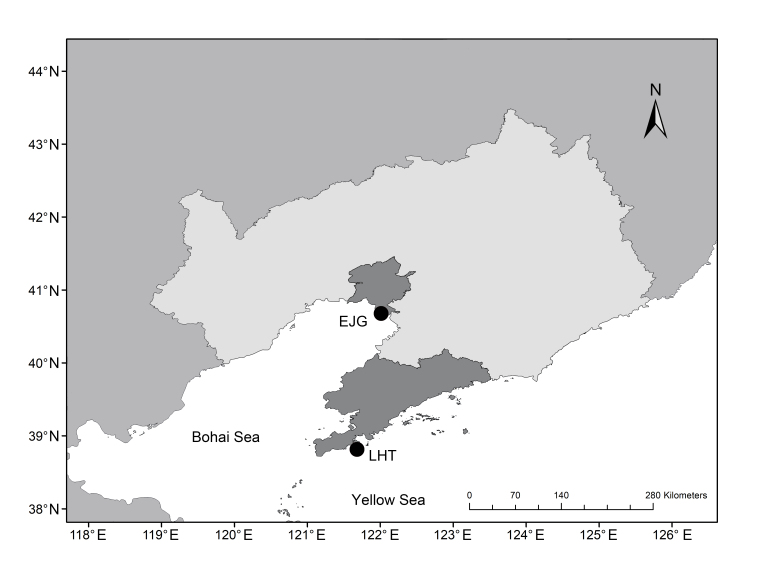
Location of sampling.
DNA identification
Genomic DNA was extracted from the tissues of six different oyster specimens, with three samples randomly selected from each locality, using the Ezup Column Animal Tissue Genomic DNA kit (Sangon Biotech, Shanghai, China) and the quality of the extracted DNA was checked using 1% agarose gel electrophoresis ( Hu et al. 2019 , Cui et al. 2021 ). The primers for the 16S rRNA gene were designed using Primer Premier 5 software. The primer sequences were as follows: 16SF (5′-CGCCTGTTTATCAAAAACAT-3′) and 16SR (5′-CCGGTCTGAACTCAGATCACGT-3′). PCR amplification was performed using the following reaction system: 1.0 µl of DNA template (50 ng/µl), 1.0 µl of 16SF primer (10 µmol/l), 1.0 µl of 16SR primer (10 µmol/l), 2.0 µl of dNTP (2.5 mmol/l), 2.0 µl of 10× buffer, 1.0 µl of Mg 2+ (25 mmol/l) and 0.1 µl of Taq (5 U/µl) and ultrapure water was added to adjust the final volume to 20 µl.
The PCR programme was as follows: pre-denaturation at 94°C for 3 min; denaturation at 94°C for 30 s, annealing at 52°C for 30 s and extension at 72°C for 1 min, repeated for 35 cycles; and a final extension at 72°C for 10 min, followed by storage at 4°C. PCR products were checked by electrophoresis. The PCR product was preliminarily detected using a gel imaging system. The DNA sequence was obtained using sequence analysis software and it was manually corrected, based on the sequence and peak charts. Amplicon purification and cycle sequencing were conducted by Sangon Biotechnology Co., Ltd., Shanghai, China.
Traditional morphological measurements
After removing surface attachments, electronic calipers (accurate to 0.01 mm) were used to measure the shell height (SH), shell length (SL) and shell width (SW) of each oyster shell. An electronic scale (accurate to 0.01 g) was used to measure the wet weight (WW), shell weight (SM) and soft tissue weight (ST) of each oyster. The data obtained from Laohutan and Erjiegou were analysed for correlation between each trait using the Pearson correlation coefficient in SPSS 26.0 software (IBM, Armonk, NY, USA). Multiple linear regression analyses were performed using oyster individual SL, SW and SH to establish the optimal multiple linear regression equation between morphological traits and quality traits in order to identify differences in oyster population morphology between the two sampling sites (Suppl. material 1 ).For this study, we examined 31 specimens of C. gigas and 26 specimens of C. ariakensis .
GM measurements
GM image acquisition was conducted as follows: After dissecting the oysters to remove the soft tissue, the right shell of each oyster was photographed with a digital camera (Canon G12, Tokyo, Japan) to capture a two-dimensional image from which data were collected. The shells were photographed in the same orientation such that the vertical line of the umbo was on the Y-axis and the disc on the same plane was parallel to the camera at the same distance. To reduce accidental errors, the photography and subsequent digitisation work were completed by one person ( Bai et al. 2014 ).
Normalisation of data processing
We used landmarks and semi-landmarks to mark and collect data from the two-dimensional images of oyster shells from two different regions. The selection of landmarks is required to reflect the morphological differences of the research objects as well as homology amongst the samples. Semi-landmarks are used to determine the overall outline of the research object more precisely. We selected 18 points, consisting of 1–6 landmarks as biological feature points of oysters and 7–18 semi-landmarks as semi-landmarks along the contour of the oyster shell. These landmarks are as follows: (1) shell apex; (2) posterior margin; (3) the widest point on the left side of the adductor muscle; (4) the widest point on the right side of the adductor muscle; (5) the widest point on the left side of the shell; (6) the widest point on the right side of the shell; (7–8) three equal points from the shell apex to the widest point on the right side of adductor muscle; (9–10) three equal points from the widest point on the right side of the adductor muscle to the widest point on the right side of the shell; (11–12) three equal points from the widest point on the right side of the shell to the posterior margin; (13–14) three equal points from the posterior margin to the widest point on the left side of the shell; (15–16) three equal points from the widest point on the left side of the shell to the widest point on the left side of the shell adductor muscle; and (17–18) three equal points from the widest point on the left side of the shell adductor muscle to the shell apex. The figure below shows the landmarks and semi-landmarks ( Valladares et al. 2010 ) (Fig. 2 ).
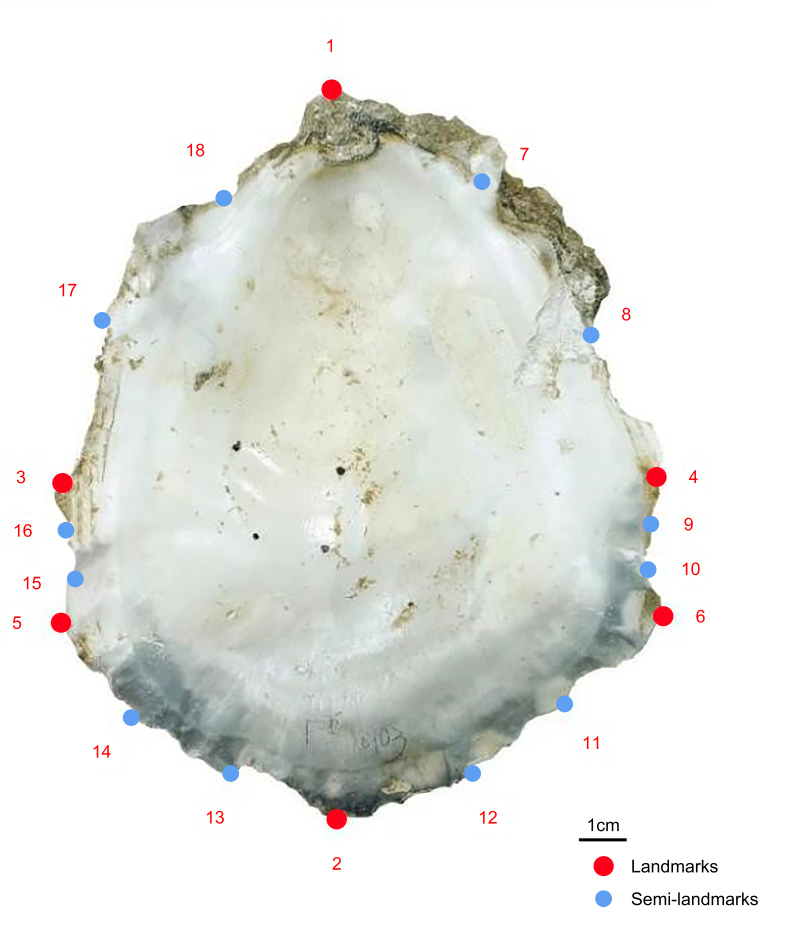
Landmarks and semi-landmarks on the oyster shell.
Red dots to represent landmarks and blue dots to represent semi-landmark.
The morphological data for the two oyster species were analysed using Generalised Procrustes Analysis (GPA) in the software Past v.3.24 ( Hammer et al. 2001 ). The analysis removed the effects of non-shell morphological differences caused by differences in shooting angles and landmark selection positions, sizes and orientations ( Wang et al. 2017 ). Subsequently, the data transformed by GPA were subjected to PCA and CVA.
DNA indentification
The 16SrRNA gene sequences of six samples from the two sampling sites were determined and the sequencing results were aligned and compared using MEGA v.7.0.26. The obtained haplotype sequences were compared with the relevant sequences downloaded from NCBI. Haplotypes for 16S rRNA sequences were identified using DnaSP 5software ( Librado and Rozas 2009 ). The Erjiegou oyster population was identified as C. ariakensis (accession number: OR598760 / OR598761 / OR598762 ) and the Laohutan oyster population was identified as C. gigas (accession number: OR598763 / OR598764 / OR598765 ) (Suppl. material 2 ).
The morphological data for the two oyster species are shown in Table 1 and Fig. 3 . For C. gigas , SH ranged from 56.19 to 159.78 mm (mean, 94.92 ± 27.91 mm), SL ranged from 23.19 to 68.55 mm (mean, 43.96 ± 11.15 mm) and SW ranged from 16.79 to 55.29 mm (mean, 29.33 ± 9.60 mm). The coefficients of variation were 29%, 25% and 33% for SH, SL and SW, respectively. For C. ariakensis , SH ranged from 78.49 to 205.64 mm (mean, 136.89 ± 32.95 mm), SL ranged from 26.28 to 114.53 mm (mean, 69.01 ± 18.63 mm) and SW ranged from 11.12 to 39.57 mm (mean, 27.85 ± 8.17 mm). The coefficients of variation were 24.07%, 26.99% and 29.32% for SH, SL and SW, respectively (Suppl. material 1 ).
Morphological measurements of the two species of oysters using traditional morphometry.
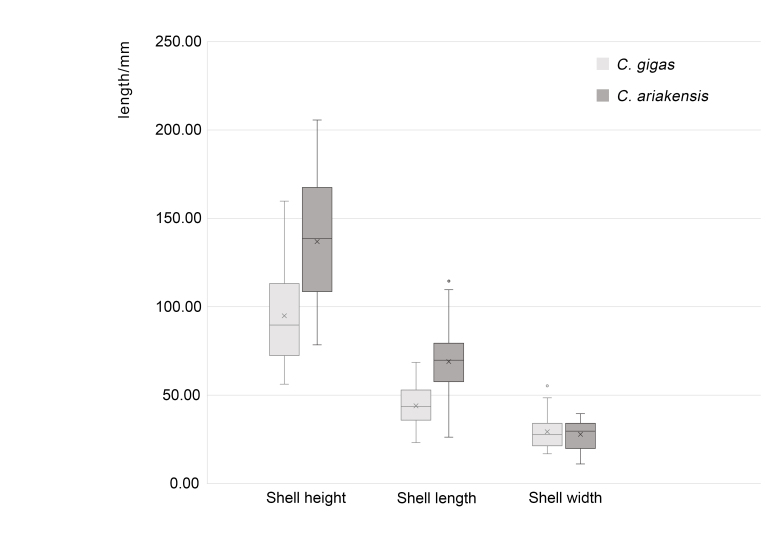
Box plot of morphometric measurements for the two oyster species.
GPA was performed on the data using the software Past v.3.24 and the resulting morphological traits of the two groups were quantified and projected on to a coordinate system to obtain a GPA overlay plot (Fig. 4 ) for the two oyster species. C. ariakensis is concentrated in the widest part of the adductor muscle and the widest part of the shell, with the widest part of the shell more prominent compared to that of C. gigas . Some points in C. ariakensis are dispersed at overlapping points. Most of the shell contour points of C. gigas are located inside those of C. ariakensis , with few scattered points and a more even distribution overall. The non-morphological factors that may have influenced the results were removed during the analysis (Suppl. material 3 ).
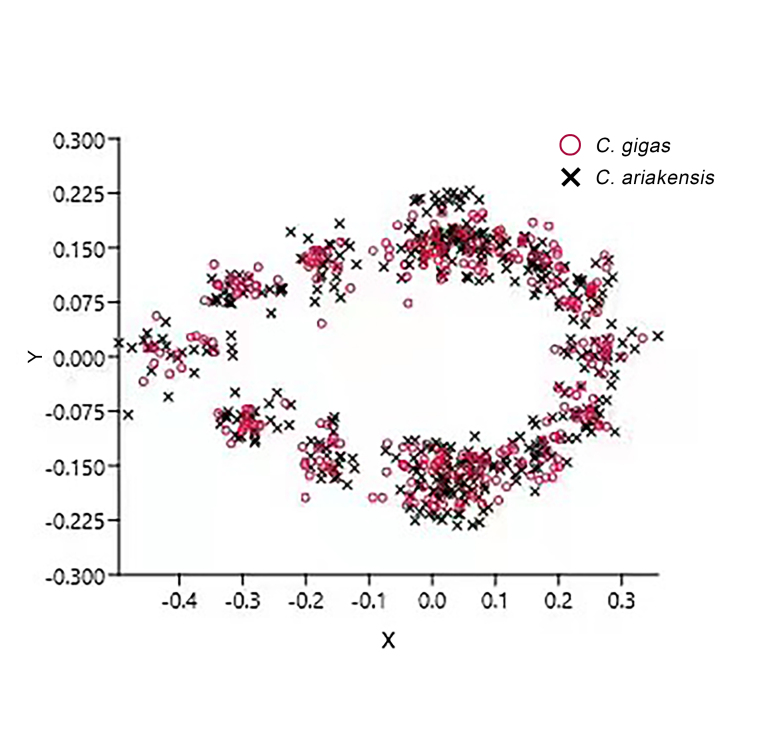
Superimposed map of the GPA of the two oyster species.
PCA and TPS
In the PCA analysis, the first three PCs together account for 78.29% of the total variance and explain the major morphological differences between C. ariakensis and C. gigas . PC1 contributes 45.22%, PC2 contributes 22.09% and PC3 contributes 10.98% to the total variance. PC1 and PC2, which together account for 85.98% of the total variance, were used as the x and y axes to create the scatter plot (Fig. 5 ). Along the positive half-axis of PC1, the shell is elongated outwards at its widest point, forming a spindle shape. Along the negative half-axis of PC1, the shell narrows inwards at its widest point, exhibiting the opposite morphology. Along the positive half-axis of PC2, the shell is elongated outwards at its widest point, whereas the ventral margin of the shell contracts inwards. Along the negative half-axis of PC2, the shell narrows inwards at its widest point, but the ventral margin expands outwards. C. ariakensis is mainly distributed in the first and third quadrants, displaying a more elongated spindle-shaped morphology. C. gigas is predominantly distributed in the second and third quadrants, exhibiting a more oval-shaped morphology. These results indicate significant morphological differences between C. ariakensis and C. gigas . The oysters in both species are mainly concentrated between PC1 axis –0.15 to 0.15 and PC2 axis –0.07 to 0.15, but the distribution of C. gigas is more concentrated, with lower variability (Fig. 5 ). C. ariakensis exhibits a larger distribution and greater variability.
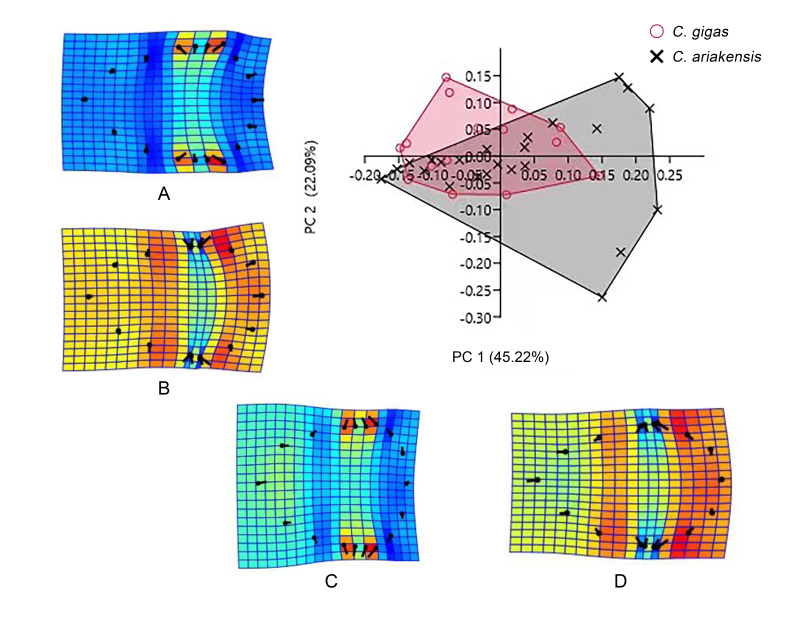
PCA and TPS analysis of the two oyster species.
The four deformation maps depicted by a thin-plate spline show the differences between the average shape and the extreme case of each PC.
TPS are deformations of a square grid, based on the differences in landmark positions between two shapes. Combining the TPS function images (A–D) revealed that the main variable points along the PC1 axis were 1, 3, 4, 5, 6, 7, 8, 9, 10, 11, 12, 13, 14, 15 and 16, while the main variable points along the PC2 axis were 2, 3, 4, 5, 6, 9, 10, 12, 13, 15 and 16. When the abscissa of the variable points changes in the positive direction along the PC1 axis, there is a tendency for the outermost edge of the shell to grow outwards and the widest part of the shell body expands outwards. When the PC1 axis changes in the negative direction, the shell body becomes shorter and the posterior edge of the shell contracts inwards. When the ordinate of the variable points changes in the positive direction along the PC2 axis, the posterior edge of the shell contracts inwards and the widest part of the shell body expands outwards. When the ordinate of the variable points changes in the negative direction along the PC2 axis, the posterior edge of the shell expands outwards, the widest part of the shell body contracts inwards and the part between the widest part of the shell body and the widest part of the oyster adductor muscle grows narrower. The distribution of the C. ariakensis in the positive direction of PC1 and the negative direction of PC2 in the figure is wider than that of the C. gigas population, indicating that C. ariakensis has a greater degree of variation in the posterior edge of the shell and the widest part of the shell body.
The results of the CVA, based on Mahalanobis distances and Procrustes distances that were calculated using the within-group covariance matrix and the between-group covariance matrix, respectively, were used to test for significant differences between predefined groups (developmental stage, sex, species) and to evaluate the reliability of classification. The Mahalanobis distance is used to represent the morphological differences between an individual and other individuals within the same population, while the Procrustes distance is used to represent the morphological differences between different groups (Fig. 6 ).
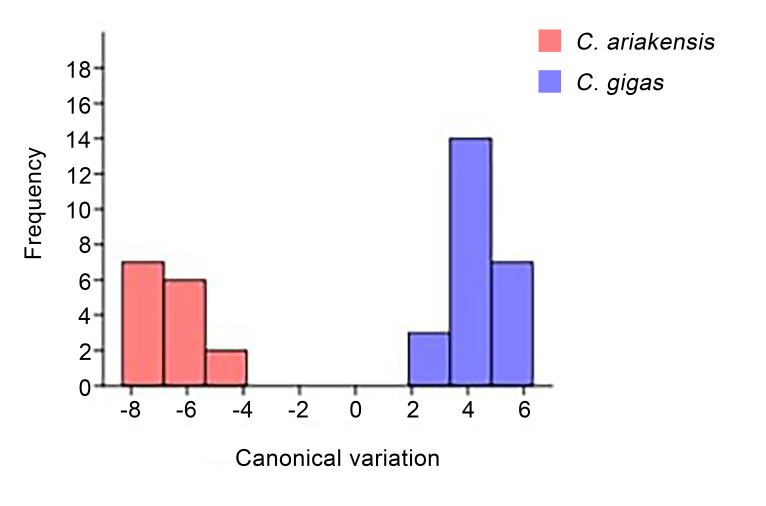
Canonical Variate Analysis (CVA) diagram of shell shapes in the two oyster species.
Figure 6 shows the results of the CVA for C. gigas and C. ariakensis . The data were imported into PAST software and the typical variable analysis histogram was plotted using morphological discriminant variables as the abscissa and sample frequency as the ordinate C. gigas and C. ariakensis are completely separated, with a discrimination rate of 100%, indicating that they can be completely separated and are two different species.
Oysters have high morphological plasticity and easily change their shell characteristics, based on the environment. Traditional classification is mainly based on shell morphology and anatomical structure, which can lead to confusion about taxonomic identification ( Littlewood 1994 ). Zhang and Lou (1956) used the classification system proposed by Hirase (1930) to categorise 25 oyster species collected along the Chinese coast into four subgenera and they provided detailed descriptions of their morphological characteristics. Harry (1985) reported that, amongst the over 100 extant oyster species recorded worldwide, nearly two-thirds have synonymous names, suggesting the existence of taxonomic inconsistencies. Naming errors and synonymous names have frequently occurred in oyster taxonomy ( Que et al. 2003 ), which has severely impacted the conservation of oyster genetic resources and the breeding of improved varieties. Molecular identification methods have significant advantages in taxonomy, as they can improve classification efficiency and accuracy. However, in practical production, their high cost prohibits non-destructive testing of large oyster populations.
In this study, we applied both traditional morphometrics and GM to analyse the morphology of C. ariakensis and C. gigas . Traditional morphometrics revealed differences in morphology between the two species. However, there was overlap between them, making it difficult to accurately identify them. GM can eliminate the effects of size, position and measurement angles by using TPS function analysis and PCA. It is a quantitative approach widely used to describe the shape of biological specimens and its covariation with other biological and environmental factors ( Zelditch et al. 2004 , Webster and Sheets 2010 ). Morphological variables are quantified using a set of Cartesian landmarks located on distinct homologous anatomical points and observed body shape variations are then displayed through user-friendly graphical representations ( Adams et al. 2004 , Zelditch et al. 2004 , Mitteroecker and Gunz 2009 ). GM is a powerful technique capable of detecting even tiny morphological differences amongst groups of specimens ( Mitteroecker and Gunz 2009 , Webster and Sheets 2010 ).
Use of these techniques revealed the presence of shell shape differences between C. ariakensis and C. gigas . Discriminant analysis, based on the typical variables, resulted in a classification accuracy of 100% for the two oyster species. This demonstrates the feasibility of using GM for oyster morphology analysis and classification. Compared to molecular methods, GM offers advantages such as speed, non-destructive sampling and the ability to analyse large sample sizes in batches. Our results provide a theoretical foundation for the future application of GM in oyster classification and seedling breeding.
GM analysis of oysters needs to be based on a large number of specimens. It can be applied to analyse and compare the shape of oyster shells or other relevant structures and to assess the effects of environmental factors or genetic variations on shell shape. By collecting landmark coordinates on the shell, researchers can quantify and compare shape differences amongst different oyster populations or individuals. This information can provide insights into the genetic diversity and adaptive strategies of oysters in different environments. GM can also be used to study the ontogenetic changes in oyster shell shape. By capturing and analysing the shape variation at different growth stages, researchers can understand how the shell shape develops and changes during an oyster's lifespan. Overall, the application of GM in oysters can contribute to our understanding of the biology, evolution and ecological interactions of oysters, as it provides a quantitative and objective approach to studying shape variations, which can lead to valuable insights in oyster research and management.
Supplementary Material
Morph data of two oysters
Data type Morphological data
File: oo_923793.xlsx
The entire sequence of two groups of oysters
Data type 16S rDNA sequence
Brief description No1-No.3 are Crassostrea ariakensis sequence No.4-No.6 are Crassostrea gigas sequence.
File: oo_923780.zip
Data type morphological data
Brief description Landmark data for two groups of oysters.
File: oo_923794.xlsx
Acknowledgements
The authors wish to express thanks to the staff of Key Laboratory of Mariculture & Stock Enhancement in North China’s Sea, Ministry of Agriculture, P.R. China for their help with the experiment. The authors are also grateful to the anonymous reviewers for the great elaboration of the manuscript through their critical reviewing and comments. In addition, the author would like to thank the International Science Editing Company for helping to improve the language content of this article.
Funding program
This study was supported by funds from Dalian Science and Technology Innovation Fund project (2021JJ12SN34), Marine Economy Development Special Project of Liaoning Province Department of Natural Resources and the National Key Research and Development Program of Dalian (2022YF16SN067).
Contributor Information
Ying Tian, Email: [email protected].
Zhenlin Hao, Email: [email protected].
Conflicts of interest
No conflict of interest to declare
Disclaimer: This article is (co-)authored by any of the Editors-in-Chief, Managing Editors or their deputies in this journal.
- Adams Dean C., Rohlf F. James, Slice Dennis E. Geometric morphometrics: Ten years of progress following the ‘revolution’. Italian Journal of Zoology. 2004;71(1):5–16. doi: 10.1080/11250000409356545. [ DOI ] [ Google Scholar ]
- Al-Kandari Manal, Oliver P. Graham, Salvi Daniele. Molecular and morphological systematics of a new, reef forming, cupped oyster from the northern Arabian Gulf: Talonostreasalpinx new species. ZooKeys. 2021;1043:1–20. doi: 10.3897/zookeys.1043.66992. [ DOI ] [ PMC free article ] [ PubMed ] [ Google Scholar ]
- Bai Ming, Yang XingKe, Wang WenCheng, Li Jing. Geometric Morphometrics, a super scientific computing tool in morphology comparison. Chinese Science Bulletin. 2014;59(10):887–894. doi: 10.1360/972012-1561. Chinese. [ DOI ] [ Google Scholar ]
- Bayne B., Anglès d'Auriac M., Backeljau T., Beninger P., Boudry P., Carnegie R., Davis J., Guo X., Hedgecock D., Krause M., Langdon C., Lapègue S., Manahan D., Mann R., Powell E., Shumway S. A scientific name for Pacific oysters. Aquaculture. 2019;499:373–373. doi: 10.1016/j.aquaculture.2018.08.048. [ DOI ] [ Google Scholar ]
- Bayne B. L., Ahrens M., Allen S. K., D'auriac M. Anglès, Backeljau T., Beninger P., Bohn R., Boudry P., Davis J., Green T., Guo X., Hedgecock D., Ibarra A., Kingsley-Smith P., Krause M., Langdon C., Lapègue S., Li C., Manahan D., Mann R., Perez-Paralle L., Powell E. N., Rawson P. D., Speiser D., Sanchez J. -L., Shumway S., Wang H. The proposed dropping of the genus Crassostrea for all Pacific cupped oysters and its replacement by a new genus Magallana: a dissenting view. Journal of Shellfish Research. 2017;36(3):545–547. doi: 10.2983/035.036.0301. [ DOI ] [ Google Scholar ]
- Bieler Rüdiger, Carter Joseph G., Coan Eugene V. Classification of bivalve families. http://zoobank.org/e1c98c0a-22b5-474a-83f9-e3e2c18f85fd . Malacologia. 2010;52(2):113–133. doi: 10.4002/040.052.0201. [ DOI ] [ Google Scholar ]
- Cui Zongmei, Hu Lisha, Li Cui, Zhang Zhen, Guo Ximing, Wang Haiyan. Identification of Saccostreamordax and a New Species Saccostrea mordoides sp. nov. (Bivalvia: Ostreidae) from China. Journal of Shellfish Research. 2021;40(1):63–75. doi: 10.2983/035.040.0107. [ DOI ] [ Google Scholar ]
- Dong Xiao Wei, Jiang Guo Liang, Li Li De, Wang Ning. Research developmentsin the general utilization of oyster. https://kns.cnki.net/kcms2/article/abstract?v=4u_pwZ3OVlCbd7227GGiCXufqyGgsahKW4DgcGgZRAPniqfo-28vS1-QlEJrpk2U-EdybpDZjpLFNeYWSytUsN9zYN25WK17uLQ3zoZTz6wiASaBU88weQEBQcDs1Pva&uniplatform=NZKPT&language=CHS . Marine Sciences. 2004;28(4):62–65. doi: 10.3969/j.issn.1000-3096.2004.04.014. Chinese. [ DOI ] [ Google Scholar ]
- Du Xin Ru, Lu Shi Bi. Anatomical study of the proxim alfemoral canal. https://kns.cnki.net/kcms2/article/abstract?v=4u_pwZ3OVlANFkChxA2QxaBqy47_ZElqTPTy96Zm3RB7Le4vpmAeaFHhQ-dvVi1n410D_hUapYJhgr7YLFlHshw4NYtMjomkjn931ahNkpZyq1d2huexzajppaM_u0x2&uniplatform=NZKPT&language=CHS Chinese Journal of Cllinical Anatomy. 2006;24(05):506–509. Chinese. [ Google Scholar ]
- Guo Ximing, Li Cui, Wang Haiyan, Xu Zhe. Diversity and Evolution of Living Oysters. Journal of Shellfish Research. 2018;37(4):755–771. doi: 10.2983/035.037.0407. [ DOI ] [ Google Scholar ]
- Hammer O., Harper D. A., D. Ryan P. PAST: Paleontological statistics software package for education and data analysis. http://palaeo-electronica.org/2001_1/past/issue1_01.htm Palaeontologia Electronica. 2001;4(1):1–9. [ Google Scholar ]
- Harry H. W. Synopsis of the supraspecific classification of living oysters (Bivalvia: Gryphaeidae and Ostreidae) https://www.biodiversitylibrary.org/item/134485 Veliger. 1985;28(2):121–158. [ Google Scholar ]
- Hirase S. Transactions: 1. On the classification of Japanese oysters. Japanese Journal of Zoology. 1930;3:1–65. [ Google Scholar ]
- Hu Lisha, Wang Haiyan, Zhang Zhen, Li Cui, Guo Ximing. Classification of Small Flat Oysters of Ostrea stentina Species Complex and a New Species Ostreaneostentina sp. nov. (Bivalvia: Ostreidae) Journal of Shellfish Research. 2019;38(2):295–308. doi: 10.2983/035.038.0210. English. [ DOI ] [ Google Scholar ]
- Jiang Xiao Dong, Cheng Yong Xu, Pan Jian Lin, LI Xiao Dong, Wu Xu Gan. Landmark-based morphometric identification of wild Eriocheirsinensis with geographically different origins. https://kns.cnki.net/kcms2/article/abstract?v=4u_pwZ3OVlDce7hNuWKMT7D0mPCkg4dgwCwsWQoeV0CR5yhx5Uqo1kyvxoW7pUh44ib8IClE5UTQsYL98ncje-j8qWQIpKmNKebmM9WpAe-uuewZh6NZh5VldX1npnAEoXQasOgTqdQ=&uniplatform=NZKPT&language=CHS Journal of Fishery Sciences of China. 2019;26(06):1116–1125. Chinese. [ Google Scholar ]
- Librado P., Rozas J. DnaSP v5: a software for comprehensive analysis of DNA polymorphism data. Bioinformatics. 2009;25(11):1451–1452. doi: 10.1093/bioinformatics/btp187. [ DOI ] [ PubMed ] [ Google Scholar ]
- Li Cui, Wang Haiyan, Guo Ximing. Classification and Taxonomic Revision of Two Oyster Species from Peru: Ostreamegodon (Hanley, 1846) and Crassostrea talonata (Li & Qi, 1994) Journal of Shellfish Research. 2017;36(2):359–364. doi: 10.2983/035.036.0208. [ DOI ] [ Google Scholar ]
- Lindberg David R., Ponder Winston F. Phylogeny and evolution of the Mollusca. University of California Press; 2008. 453. [ DOI ] [ Google Scholar ]
- Littlewood D. T.J. Molecular phylogenetics of cupped oysters based on partial 28S rRNA gene sequences. Molecular Phylogenetics and Evolution. 1994;3(3):221–229. doi: 10.1006/mpev.1994.1024. [ DOI ] [ PubMed ] [ Google Scholar ]
- Miller Jonathan P. Geometric morphometric analysis of the shell of Cerionmumia (Pulmonata: Cerionidae) and related species. Folia Malacologica. 2016;24(4):239–250. doi: 10.12657/folmal.024.020. [ DOI ] [ Google Scholar ]
- Minton Russell L., Norwood Andrew P., Hayes David M. Quantifying phenotypic gradients in freshwater snails: a case study in Lithasia (Gastropoda: Pleuroceridae) Hydrobiologia. 2008;605(1):173–182. doi: 10.1007/s10750-008-9332-1. [ DOI ] [ Google Scholar ]
- Mitteroecker Philipp, Gunz Philipp. Advances in geometric morphometrics. Evolutionary Biology. 2009;36(2):235–247. doi: 10.1007/s11692-009-9055-x. [ DOI ] [ Google Scholar ]
- Perez Kathryn E. A new species of Praticolella (Gastropoda: Polygyridae) from northeastern Mexico and revision of several species of this genus. https://www.biodiversitylibrary.org/item/203168 Nautilus. 2011;125(3):113–126. [ Google Scholar ]
- Que Hua Yong, Liu Xiao, Wang Hai Yan, Zhang Su Ping, Zhang Guo Fan, Zhang Fu Sui. Systematics of oysters along the coast of China: Status and countermeasures. http://en.cnki.com.cn/Article_en/CJFDTOTAL-BIRD200304023.htm Chinese Journal of Zoology. 2003;38(4):110–113. Chinese. [ Google Scholar ]
- Rohlf F. James, Slice Dennis. Extensions of the procrustes method for the optimal superimposition of landmarks. Systematic Zoology. 1990;39(1):40–59. doi: 10.2307/2992207. [ DOI ] [ Google Scholar ]
- Ruppert E. E. Morphology of Hatschek's nephridium in larval and juvenile stages of Branchiostomavirginiae (Cephalochordata) https://www.tandfonline.com/doi/abs/10.1080/00212210.1996.10688879 Israel Journal of Zoology. 2013;42(Suppl 1):161–182. [ Google Scholar ]
- Salvi Daniele, Macali Armando, Mariottini Paolo. Molecular phylogenetics and systematics of the bivalve family Ostreidae based on rRNA sequence structure models and multilocus species tree. PLOS One. 2014;9(12):e116014. doi: 10.1371/journal.pone.0108696. [ DOI ] [ PMC free article ] [ PubMed ] [ Google Scholar ]
- Shu Yu, Shi Ling, Hao Zhen Lin, Mao Jun Xia, Wang Xu Bo, Tian Ying, Chang Ya Qing. Application of geometric morphology to the morphological classification and phylogeny of eight of scallop species. Marine Sciences. 2022;46(06):61–69. doi: 10.11759/hykx20210427003. Chinese. [ DOI ] [ Google Scholar ]
- Su Wei, Song Yi Gang, Qi Min, Du Fang. Leaf morphological characteristics of section Quercus based on geometric morphometric analysis. Chinese Journal of Applied Ecology. 2021;32(07):2309–2315. doi: 10.13287/j.1001-9332.202107.001. Chinese. [ DOI ] [ PubMed ] [ Google Scholar ]
- Valladares Alejandro, Manríquez Germán, Suárez-Isla Benjamín A. Shell shape variation in populations of Mytiluschilensis (Hupe 1854) from southern Chile: a geometric morphometric approach. Marine Biology. 2010;157(12):2731–2738. doi: 10.1007/s00227-010-1532-3. [ DOI ] [ Google Scholar ]
- Wang He Kun Yuan, Tang Yong Tao, Li Guo Gang, Zhang Ren Yi, Feng Chen Guang, Tong Chao, Liu Si Jia, Zhang Cun Fang, Tian Fei, Zhao Kai. Geometric morphometrics of the cephalic contour and its morphological variations among schizopygopsis stoliczkai (teleostei: cyprinidae) https://kns.cnki.net/kcms2/article/abstract?v=4u_pwZ3OVlAobwVQo63b6pXJNOjGVh-JGOl3QGoPaF11QuvthzLiWU1dgUhLY4SBIawQJBhMdcHXvF124RO-zfLDZgUgVQ_vLQQDSgI0dOJvlgcu22O05CUK9-F3JR6LENmccT4IW7Y=&uniplatform=NZKPT&language=CHS Acta Hydrobiologica Sinica. 2017;41(1):182–193. Chinese. [ Google Scholar ]
- Wang Hai Yan, Guo Xi Min, Liu Xiao, Zhang Su Ping, Xu Feng Shan, Zhang Guo Fan. Classification study of common oysters in northern coastal China. https://kns.cnki.net/kcms2/article/abstract?v=4u_pwZ3OVlCcqWjY8Pi3VqmjD5a_oiVW05IGUQXYJzrCNUu_qW9Jbhhero2BAzZqKk20gdmO-FuMeW8J4KtBc2NkEtAwb0tSupBKEz6uKvmO5eJ_2QYwqcrCTNid-JthqI9hxySF5G8=&uniplatform=NZKPT&language=CHS 13th Symposium of Chinese Society of Malacology, Chinese Society for Oceanology and Limnology. 2007 Chinese.
- Webster Mark, Sheets H. David. A practical introduction to landmark-based geometric morphometrics. The Paleontological Society Papers. 2010;16:163–188. doi: 10.1017/s1089332600001868. [ DOI ] [ Google Scholar ]
- Wijsman J. W. M., Troost K., Fang J, Roncarati A. In: Goods and Services of Marine Bivalves. Smaal A. C., et al., editors. Springer; 2018. Global production of marine bivalves.591. [ DOI ] [ Google Scholar ]
- Wu Qi Sheng, Wang Xiao Qing, Zeng Zhi Nan, Ning Yue. Advance in taxonomic of oysters in China. https://kns.cnki.net/kcms2/article/abstract?v=4u_pwZ3OVlA6-eQKLEHGzOGcG7ef41TuH_p4uHsafoFc9M_WfHj3ZOJsd2yE2hB1u6pGawvNntN5_2vwMs-NtfjC3x83vGE1UkpobwUgBvhKbBd_nS34DwEOQJ92vdQ7&uniplatform=NZKPT&language=CHS Journal of Fujian Fisheries. 2011;33(01):67–72. Chinese. [ Google Scholar ]
- Xu Feng Shan. Bivalve mollusca of China seas. Beijing Science and Technology Press; 1997. 333. Chinese. [ Google Scholar ]
- Xu Feng Shan, Zhang Su Ping. An illustrated Bivalvia Mollusca fauna of China Seas. Beijing Science and Technology Press; 2008. 336. Chinese. [ Google Scholar ]
- Zelditch M. L., Swiderski D. L., Sheets H. D. Geometric morphometrics for biologists. Elsevier Academic Press; 2004. 443. [ DOI ] [ Google Scholar ]
- Zhang Xi, Lou Zi Kang. A study of Chinese oysters. https://kns.cnki.net/kcms2/article/abstract?v=4u_pwZ3OVlCuwrDJzipIOoLvg6pnNi-0rVZpnsovnD2yKKvboJVoV_jMDNzqfD8qM5qXYIRZy70CmimP3P3Oce4_cFnRbfjnaK_XywGo-sZuje9J80JBxe42GeXFWRWZ&uniplatform=NZKPT&language=CHS Current Zoology. 1956;8(1):65–94. Chinese. [ Google Scholar ]
Associated Data
This section collects any data citations, data availability statements, or supplementary materials included in this article.
Supplementary Materials
- View on publisher site
- PDF (646.3 KB)
- Collections
Similar articles
Cited by other articles, links to ncbi databases.
- Download .nbib .nbib
- Format: AMA APA MLA NLM
Add to Collections
We can help you reset your password using the email address linked to your BioOne Complete account.

- BioOne Complete
- BioOne eBook Titles
- By Publisher
- About BioOne Digital Library
- How to Subscribe & Access
- Library Resources
- Publisher Resources
- Instructor Resources
- FIGURES & TABLES
- DOWNLOAD PAPER SAVE TO MY LIBRARY
Oysters are difficult to classify because of plasticity in shell morphology. Difficulties in classification have hindered the understanding of oyster diversity and evolution. Recent molecular studies of living oysters have revealed high genetic diversity at species, population, and genome levels. New and cryptic species have been discovered, revealing surprisingly high species diversity under similar shell morphology. Genetic analyses have identified several species complexes where low genetic divergence indicates recent or ongoing speciation during the past 3–4 million years. Ongoing speciation is also supported by exceptionally high population divergence within some species. The oyster genome is highly polymorphic and gene-rich, with extensive expansion of genes related to stress and immune responses. High levels of genetic diversity and gene expansion in oysters are likely adaptations to variable environments. Local adaptation in oysters may be pervasive but countered by strong gene flow and balancing or multidirectional selections that favor diversity. Oysters have experienced several expansion and contraction events because of climate change since their origin in Permian. Studies on molecular adaptations to recent and historic climate change may provide insights into the evolution and speciation of oysters.

KEYWORDS/PHRASES
Publication title:, collection:, publication years.
- Open access
- Published: 07 January 2023
A remarkably diverse and well-organized virus community in a filter-feeding oyster
- Jing-Zhe Jiang 1 , 2 , 3 , 4 na1 ,
- Yi-Fei Fang 2 na1 nAff5 ,
- Hong-Ying Wei 2 , 6 ,
- Peng Zhu 2 ,
- Min Liu 2 ,
- Wen-Guang Yuan 3 ,
- Li-Ling Yang 4 ,
- Ying-Xiang Guo 4 ,
- Tao Jin 6 ,
- Mang Shi 7 ,
- Tuo Yao 1 ,
- Ling-Tong Ye 1 ,
- Shao-Kun Shi 8 ,
- Meng Wang 9 ,
- Ming Duan 10 &
- Dian-Chang Zhang 1
Microbiome volume 11 , Article number: 2 ( 2023 ) Cite this article
5518 Accesses
5 Altmetric
Metrics details
A Correction to this article was published on 09 February 2023
This article has been updated
Viruses play critical roles in the marine environment because of their interactions with an extremely broad range of potential hosts. Many studies of viruses in seawater have been published, but viruses that inhabit marine animals have been largely neglected. Oysters are keystone species in coastal ecosystems, yet as filter-feeding bivalves with very large roosting numbers and species co-habitation, it is not clear what role they play in marine virus transmission and coastal microbiome regulation.
Here, we report a Dataset of Oyster Virome (DOV) that contains 728,784 nonredundant viral operational taxonomic unit contigs (≥ 800 bp) and 3473 high-quality viral genomes, enabling the first comprehensive overview of both DNA and RNA viral communities in the oyster Crassostrea hongkongensis . We discovered tremendous diversity among novel viruses that inhabit this oyster using multiple approaches, including reads recruitment, viral operational taxonomic units, and high-quality virus genomes. Our results show that these viruses are very different from viruses in the oceans or other habitats. In particular, the high diversity of novel circoviruses that we found in the oysters indicates that oysters may be potential hotspots for circoviruses. Notably, the viruses that were enriched in oysters are not random but are well-organized communities that can respond to changes in the health state of the host and the external environment at both compositional and functional levels.
Conclusions
In this study, we generated a first “knowledge landscape” of the oyster virome, which has increased the number of known oyster-related viruses by tens of thousands. Our results suggest that oysters provide a unique habitat that is different from that of seawater, and highlight the importance of filter-feeding bivalves for marine virus exploration as well as their essential but still invisible roles in regulating marine ecosystems.
Video Abstract
As the most abundant biological entities on Earth, viruses can infect organisms from every phylum. They play critical roles in host mortality, metabolism, physiology, and evolution, impacting marine biogeochemical cycling and shaping the Earth’s microbiomes [ 27 , 104 , 92 ]. Culture-independent next-generation sequencing technologies have recently been used to explore the tremendous diversity of the virosphere from multiple samples [ 13 , 59 , 60 , 66 , 84 , 85 ]. Among the findings, progress in the discovery of marine viruses (mainly phages of marine bacteria) is particularly impressive [ 12 ], including the creation of a global ocean DNA virome 2.0 (GOV 2.0) dataset, which contains 195,728 viral populations detected from 145 seawater samples collected worldwide [ 31 ].
Many studies have focused on free viruses in seawater, whereas viruses in marine animals have been largely neglected. Marine animals are teeming with viruses that inhabit hosts’ surfaces, body spaces, and blood [ 82 ]. Virome of marine animals form connections with their host, which is vital to the interaction of the microbe community both in and outside the host’s body [ 2 , 30 ].
Bivalves of the phylum Mollusca (i.e., oysters, mussels, scallops, and clams) represent the largest number of described marine animal species and they are known to play vital roles in the functioning of marine ecosystems. Many bivalves are important fishery and aquaculture species as well as models for studying ocean acidification, biomineralization, and adaptation to coastal environments under climate change [ 52 , 73 , 106 ]. Some sedentary bivalves, such as oysters and mussels, impose a stabilizing and enduring ecological effect on a given area. However, their population characteristics, which include high roost numbers and species co-habitation, provide ideal conditions for the transmission of viruses with the water flow. Importantly, as filter-feeding animals, bivalves can draw up to 5 L of water per hour through their gills and thereby concentrate suspended microbes and particles by factors of a thousand to a hundred-thousand times the concentrations found in seawater [ 5 , 65 ]. Indeed, the enrichment of human enteric viruses [ 61 ] and mimiviruses [ 1 ] in oyster gill or gut tissues is clearly an effect of their filter-feeding habit.
Bivalves have a semi-open circulatory system and lack body segmentation,their hemolymph is pumped into a cavity (hemocoel) and the material in it is directly exchanged between the blood and body cells. Consequently, it is interesting to speculate on the microbial communities present in bivalves. Previous studies have shown that the microbiota in oysters is mainly affected by the external environment and by disturbances [ 55 , 56 , 63 , 99 ], although the internal microbial community can differ significantly from the microbiota in the ambient water. This indicates that the internal environment of the oyster has a selective effect on the microbiota that it hosts [ 88 , 54 ]. To date, few studies have reported on the viral microbial community in oysters [ 22 ]. Whether bivalves provide a similar environment or a unique habitat for marine viruses and whether bivalves spread viruses and regulate coastal microbial communities are important questions yet to be answered.
Oysters of the family Ostreidae are widely distributed in the intertidal zone globally and are possibly the most highly produced seafood in the world. China is the largest producer of oysters, accounting for 85.3% of the world’s total production (FAO, 2019). Here, we report an extensive Dataset of Oyster Virome (DOV) that consists of 54 sequencing libraries from different tissues, sampling sites, and sampling times of Crassostrea hongkongensis , the most farmed species of oyster along the south coast of China. We used virus-like particle (VLP) enrichment and targeted amplification strategies and thereby built a ‘knowledge landscape’ of the oyster virome community, its function, and the factors influencing both RNA and DNA viruses, which provides a good foundation to address questions about the connections between bivalves and marine viruses.
Material and methods
Oyster sampling.
The oyster samples in this study were all adults of C. hongkongensis and the sample collection spanned 5 years, from June 2014 to July 2019. We divided the samples into nine time batches according to the chronological order of collection (Table S 1 : Time_Batch_ID, Sampling_Date). In addition, the samples were divided into four other groups. Amplification groups were based on the amplification method: whole genome amplification (WGA), whole transcriptome amplification (WTA), reverse transcription and WGA (RT-WGA), or double-stranded DNA (dsDNA) (Table S 1 : Amplification_Method). Tissue groups were based on the tissue origin (i.e., mixed tissues and hemolymph of adults) (Table S 1 : Tissue_Origin). Site groups were based on the sampling site (BH, HD, LJ, SZ, TS, YJ, and ZH) (Fig. 1 D) (Table S 1 : Sampling_Site). Finally, status groups were based on the health status of the oyster (i.e., apparently healthy or moribund) (Table S 1 : Health_Status). The designation “healthy” denotes that there was no large-scale death of farmed oysters before or after sampling and that normal and fleshy individuals were collected. The designation “moribund” indicates that large-scale mortality was taking place at the time of sampling, and consequently, surviving but moribund individuals were collected. In total, we constructed 54 sequencing libraries (Table S 1 : Library_ID) with 35 samples (Table S 1 : Sample_ID).
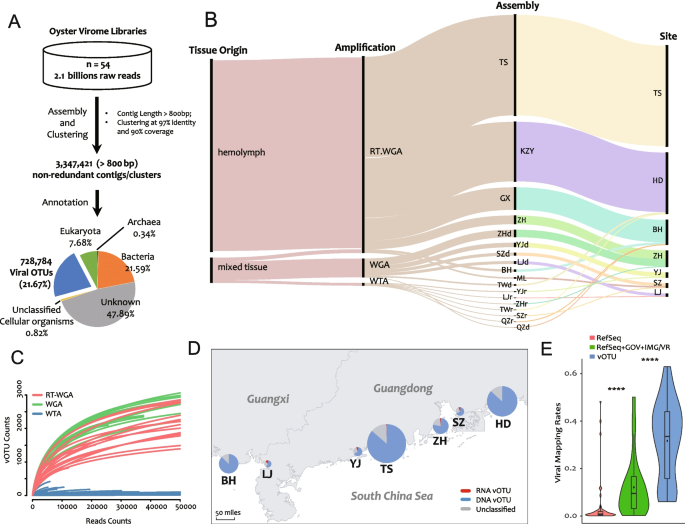
Overview of the Dataset of Oyster Virome (DOV). A De novo assembly and annotation pipeline. B Sankey diagram of the relationship among different batches and groups. The heights of the black vertical bars proportionally represent the number of viral contigs (vOTUs) assembled under the each group. C Rarefaction curves of the oyster viromic libraries. RT-WGA, reverse transcription and whole genome amplification; WGA, whole genome amplification; WTA whole transcriptome amplification. D Sampling site distribution map and the number of detected vOTUs from each site. The radius of the pie chart indicates the number of DNA, RNA, and unclassified vOTUs. E Mapping rates of viral reads in total clean reads. RefSeq, NCBI viral RefSeq genomes (release March 2021); GOV (release July 2020), Global Ocean Virome dataset; IMG/VR (release January 2018), a database of cultured and uncultured DNA viruses and retroviruses maintained by the Joint Genome Institute; vOTU, de novo assembled vOTUs in the DOV. “****” indicates p < 0.0001 (Student’s t -test between the three mapping rates)
Time batch 1 (dCh) comprised dying animals collected from an oyster farming area at Beihai (BH), Guangxi Province, in June 2014. Time batch 2 included 3 samples (ChYJa–c) collected from an oyster farming area at Yangjiang (YJ), Guangdong Province, in September 2015. Time batch 3 comprised 12 samples (QZa–c, TWa–c, ZHa–c, and LJa–c) that were separately collected from oyster farming areas in the Qinzhou area (QZ) of BH, the Tanwei area (TW) of Huidong (HD), and at Zhuhai (ZH) and Lianjiang (LJ) in Guangdong Province in November 2015. Time batch 4 comprised 3 samples (SZa–c) collected from the Shenzhen (SZ) oyster farming area in Guangdong Province in April 2016. Time batch 5 comprised 3 samples (ML-1–3) collected at SZ in May 2016. Time batch 6 contained 2 moribund samples (BHos1–2) collected in BH in July 2016. Time batch 7 comprised 9 samples (GX, K1ZY, K2ZY, T2S, T4S, T5S, T6S, T8S, and ZH) which were separately collected from BH, Kaozhouyang (K#ZY) of Huidong (HD), Taishan (T#S), and ZH in Guangdong Province in May 2017; of these, samples K1ZY, K2ZY, and T8S were healthy, and the others were moribund. Time batch 8 (os) were oysters collected in July 2018. The samples in time batches 1–8 were collected and preserved by the South China Sea Fisheries Research Institute (Guangdong, China). Time batch 9 (HS) were oysters purchased in July 2019 from the Huangsha (HS) Aquatic Product Market in Guangzhou, Guangdong Province, but their original farming location was ZH. The samples in that batch were collected and preserved by Guangdong Magigene Biotechnology Co., Ltd (Guangzhou, China). Details on the total samples are given in Table S 1 .
For time batches 1–6 and 8, the tissues (including gills, mantle, and hepatopancreas) from three adult individuals were mixed to form single samples. For time batch 7, a 1-mL syringe was used to draw hemolymph from the pericardial cavity of the individuals, and then 5–8 individuals were mixed to form single samples. The tissue and hemolymph samples ( n = 35) were all quickly frozen in liquid nitrogen, temporarily stored with dry ice during transportation, and placed in an ultra-low-temperature freezer at − 80 °C for long-term storage.
VLP enrichment
All 35 samples were processed to enrich for VLPs as described by Wei et al. [ 100 , 101 ] and using the online protocols ( https://doi.org/10.17504/protocols.io.m4yc8xw ). First, 500 mg of mixed tissue (including gills, mantle, and hepatopancreas) was dissected and ground to powder in liquid nitrogen. The powder was further homogenized in approximately 2–5 volumes of sterile SB buffer (0.2 M NaCl, 50 mM Tris–HCl, 5 mM CaCl2, 5 mM MgCl2; pH 7.5). After three rounds of freezing and thawing, the pellets were resuspended entirely in 10 volumes of pre-cooled SB buffer. For the hemolymph sample, 10 mL hemolymph was mixed with an equal volume of 2 × SB buffer and then directly subjected to three rounds of freezing and thawing. The following steps were the same for the tissue and hemolymph samples. All the samples were centrifuged at 1000, 3000, 5000, 8000, 10,000, and 12,000 × g for 5 min each at 4 °C using a 3K30 centrifuge (Sigma, Osterode am Harz, Germany), and the supernatants were retained. Cell debris, organelles, and bacterial cells were further removed using a Millex-HV filter with 0.22-μm pore size. The filtrates were transferred to ultracentrifuge tubes containing 28% (w/w) sucrose using a syringe. The tubes were transferred to an ice bath for 10 min before centrifugation in a Himac CP 100WX ultracentrifuge (Hitachi, Tokyo, Japan) at 300,000 × g for 2 h. Supernatants were discarded and the precipitates were fully resuspended in 720 μL of water, 90 μL 10 × DNase I Buffer, and 90 μL DNase I (1 U/μL) and then incubated at 37 °C with shaking for 60 min, followed by storage overnight at 4 °C, before being transferred to 2-mL centrifuge tubes.
Viral nucleic acid extraction and amplification
Total nucleic acid was extracted from the VLPs using an HP Viral DNA/RNA Kit (R6873; Omega Bio-Tek, Norcross, GA, USA); carrier RNA was not used, to avoid potential interference with sequencing results. A Qubit™ dsDNA HS Assay Kit (Q32851) and Qubit™ RNA HS Assay Kit (Q32855) (Thermo Fisher Scientific, Waltham, MA, USA), respectively, were used to quantify the concentrations of dsDNA and RNA.
Virome studies are highly reliant on amplification because the viral biomass in natural samples is very low [ 4 , 71 ]. Because most available amplification methods introduce bias, it is challenging to study viromic sequencing data quantitatively [ 23 , 68 ]. Here, a REPLI-g Cell WGA & WTA Kit (150052; Qiagen, Hilden, Germany), which is based on the multiple displacement amplification (MDA) method, was used to uniformly amplify the whole genomes (WGA) and whole transcriptomes (WTA) [ 35 , 67 , 70 ]. MDA has many significant advantages over other amplification methods, such as replicating up to 70 kb, more-even coverage, and 1000-fold higher fidelity than Taq polymerase amplification [ 35 , 87 ], which make MDA widely used in virome studies.
We used WGA and WTA to construct libraries in four batches of mixed tissues, which accounted for 70% (38/54) of all libraries (Table S 1 ). To better compare the RNA and DNA virus communities, we specifically compared differences in the viral communities obtained with the two amplification methods using the same batches of samples ( n = 18) (Table S 1 : Time_Batch_ID #2–4) at the same time. RT-WGA is a modified protocol that simultaneously amplifies DNA and RNA [ 49 , 101 ]. In this study, 14 libraries were constructed based on RT-WGA, including hemolymph and mixed tissue samples (Table S 1 ). The main reason for using RT-WGA is to simultaneously detect both DNA and RNA potential viral pathogens in diseased batches (Table S 1 : Time_Batch_ID #6 and #7), for the sake of cost efficiency. The steps for the WGA, WTA, and RT-WGA methods were according to the online protocols ( https://doi.org/10.17504/protocols.io.m5vc866 ). For WTA, there is a “DNA wipeout” step before reverse transcription that aims to remove DNA altogether, but this step is not part of the WGA and RT-WGA protocols. Compared with the protocols of WTA and RT-WGA, the WGA protocol skips the reverse transcription reaction to avoid amplifying RNA in the downstream reaction. In addition, two other samples were directly subjected to random shotgun library preparation using a Nextera XT DNA Library Preparation Kit (Illumina) following the manufacturer’s protocol. Because of the limited data quality and sample number, these two libraries were not included in the following analysis of virus diversity.
Library construction and sequencing
Amplified DNA was quantified by gel electrophoresis and Nanodrop 2000 spectrophotometer (Thermo Fisher Scientific) and randomly sheared by ultrasound sonication (Covaris M220) to produce fragments of ≤ 800 bp. The sticky ends were repaired, and adapters were added using T4 DNA polymerase (M4211, Promega, USA), Klenow DNA polymerase (KP810250, Epicentre), and T4 polynucleotide kinase (EK0031, Thermo Fisher Scientific, USA). Fragments of 300–800 bp were collected after electrophoresis. After amplification, libraries were pooled and subjected to 150-bp, 250-bp, or 300-bp paired-end sequencing on the NovaSeq 6000, HiSeq X Ten, and MiSeq platforms (Illumina, USA). Considering that the RT-WGA libraries were likely to have higher virus diversity than the WGA and WTA libraries [ 100 ], they were sequenced with higher depth and thus produced better assembly results (Table S 1 ).

Virus detection and quantification based on reference viral genomes
Instead of using the traditional read alignment tools such as BLAST, BWA, and Bowtie2, we used FastViromeExplorer [ 94 ], a pipeline developed for fast and accurate virus detection and quantification in metagenomics data. FastViromeExplorer filters the alignment results based on minimal coverage criteria and the minimal number of mapped reads and accurately reports virus types and relative abundances. The program Kallisto v0.43.1, integrated with FastViromeExplorer, was used with the default settings to map clean reads against three reference databases: the National Center for Biotechnology Information (NCBI) Reference Sequence (RefSeq) database, Global Ocean Virome database (GOV) [ 80 ], and the Integrated Microbial Genome/Virus (IMG/VR) system, separately, to generate a reference abundance table. The RefSeq database (March 2021 update) contained 14,042 viral genomes or genome segments; GOV [ 80 ] included 298,383 epipelagic and mesopelagic viral contigs; and IMG/VR contained 125,842 metagenomic viral contigs of the set of sequences collected from the Joint Genome Institute’s Earth Virome project [ 66 ].
Virus detection and quantification based on de novo assembly (vOTU annotation)
High-quality clean reads were trimmed using fastp v0.20.0 [ 17 ] (options: –correction, –trim_poly_g, –trim_poly_x, –overrepresentation_analysis, –trim_front1 = 16, –trim_tail1 = 2, and –length_required = 50), and reads that matched the Illumina sequencing adapters were removed (option: –detect_adapter_for_pe). The clean reads in libraries that were in the same assembly group were pooled and assembled using MEGAHIT v1.2.9 [ 51 ] with the default settings. Only contigs longer than 800 bp were kept. To detect low-abundance contigs, clean reads that did not map back to the first round of assembled contigs were reassembled for two additional rounds, and then all remaining reads were pooled and assembled together. Contigs from all four assembly rounds were pooled and clustered at 97% global average nucleotide identity with at least 90% overlap of the shorter contig using cd-hit-est v4.8.1 (options: -aS 0.9 -c 0.97 -G 1 -M 0 -T 0 -g 1) [ 50 ], resulting in 3,347,421 nonredundant contigs (Fig. 1 A).
The nonredundant contigs were annotated using Diamond v0.9.24.125 (options: -e 1e-10, –max-target-seqs 50) against the NCBI nr database (March 2021 release). Among them, 728,784 (21.77%) of the total contigs were annotated as the viral origin (i.e., vOTUs); 7.68% were Eukaryota, 0.34% were Archeae, 21.59% were bacteria, 0.82% were unclassified cellular organisms, and 47.89% were of unknown origin (Fig. 1 A). FastViromeExplorer was used with the default settings to map the clean reads against the vOTU contigs to obtain the vOTU abundance table.
Viral genome integrity, taxonomy, and auxiliary metabolic gene analysis
The viral genome completeness of assigned contigs was tested using CheckV v0.7.0 and its associated database [ 59 , 60 ]. After removing false-positive contigs that matched more host genes than viral genes, 3,473 nearly complete viral genomes were obtained.
Three methods (Diamond, vContact2, and PhaGCN) were used to determine the taxonomy of the viral contigs at the family level. Diamond annotations were further processed using two scripts (daa2rma and rma2info) in MEGAN6 [ 38 ] with default parameters, and parsed to taxonomy annotations. The advantage of Diamond is that there is no minimum length requirement for query sequences; however, it has three drawbacks: low accuracy, low annotation rates, and inaccurate taxonomy of NCBI. PhaGCN is a novel semi-supervised learning model that combines the strengths of a BLAST-based model and a learning-based model using a knowledge graph [ 83 ]. For comparison purposes, only vOTUs of > 10 kb were compared using PhaGCN and vContact2 with default parameters.
To mine the auxiliary metabolic genes (AMGs) from DOV, Vibrant v1.2.1 [ 45 ] was used. Salmon v1.5.2 [ 69 ] was used with default settings to map clean reads against the AMG dataset to obtain the AMG abundance table.
Viral contamination assessment
The experimental preparation for viromic sequencing involves the use of various reagents, many of which have been proved to carry contaminated viral sequences of unknown origin [ 32 ]. The extent of viral contamination in common laboratory components, especially viruses with small single-stranded DNA (ssDNA) genomes, has been reported previously [ 3 , 72 ].
To assess the viral contaminant level in this study, all the 3,347,421 nonredundant contigs (≥ 800 bp; not only viral contigs) were used as queries in a BLASTN search (with the parameters set as 95% identity and 95% query coverage) against the approximately 500 contaminant viral sequences reported by Asplund et al. [ 3 ] and Porter et al. [ 72 ]. We found little evidence of viral contamination, no sequences matched with 100% identity, no expected circoviruses or RNA viruses were detected, and most of the alignments were with dsDNA phages (Additional file 1 ). The 3473 near-complete viral genomes were used as queries in the same BLASTN search, but no matches were found. We also used Salmon v1.5.2 to map all the clean reads in the DOV libraries to the contaminant viral sequences. The mapping rates for most of these libraries were < 0.01% (Additional file 2 ), which is consistent with the BLASTN results.
Viral community and statistical analysis
In this study, the transcripts per million (TPM) value was used to represent the relative abundance of the reference viral genomes, vOTUs, and AMGs. Based on the TPM-transformed abundance table, R and Excel were used to analyze the corresponding viral diversity and community structures. The vegan and ggplots R packages were used to calculate α-diversity indexes and plot the nonmetric multidimensional scaling (NMDS). Analysis of variance (ANOVA) and Tukey’s HSD were used to test the differences between groups, with the significance level set at 0.05. For the Procrustes analysis, the characteristic axis coordinates of NMDS were extracted as the input of the Procrustes function, and the protest function was used to perform the substitution test to evaluate the significance of the results. All the figures in this study were output using basic plotting tools (including R v4.2.1, Gephi v0.9, and iTol v6) and Excel and finally combined and adjusted in Adobe Illustrator CC.
Results and discussion
Overview of the dataset of oyster virome (dov).
For this study, we used 35 samples of mixed tissue or hemolymph from Crassostrea hongkongensis collected at nine time points and from seven major oyster farming areas along the south coast of China (Fig. 1 ; Table S 1 ). Fifty-four oyster virome libraries were constructed using three primary amplification methods (WTA, WGA, and RT-WGA) and then sequenced (Table S 1 ). A total of 3,347,421 nonredundant contigs (of ≥ 800 bp) were obtained after assembly. Among them, 728,784 (21.77%) were annotated as viral origin by comprehensive blast (Fig. 1 A), which we called the DOV. The viral contigs were assembled mainly from the RT-WGA libraries of hemolymph samples with higher sequencing coverages (Fig. 1 B). Rarefaction curves (Fig. 1 C) show that the sequencing depths were valid, and the vOTU numbers in the WTA libraries were the lowest among all the libraries.
Notably, the ratio of viral reads (mapping rate) varied greatly depending on the reference databases that were searched (Fig. 1 E). The mapping rate of de novo assembled vOTUs (29.81%) was much higher than the mapping rates of the RefSeq (NCBI viral reference genomes) (3.50%) and the RefSeq plus two other public virus datasets, GOV and IMG/VR (12.06%) (Fig. 1 E; Table S 1 ). The higher mapping rates of vOTUs confirmed that the VLP enrichment protocol was effective [ 53 , 101 ], indicating that filter-feeding oysters can efficiently accumulate environmental viruses. The low mapping rates of the reference genomes (3.50% and 12.06%) imply that the viruses found in the oysters were largely previously unknown. To our knowledge, this is the biggest viral metagenomic dataset currently available for any marine animal.
Viruses in oysters
Compared with the extensive studies of marine DNA viruses, investigations of oyster-related virus have focused mainly on transcriptomic data and RNA viruses. Rosani et al. [ 75 – 77 ] assembled 26 novel and nearly complete RNA virus genomes from the public transcriptomic data of C. gigas and C. corteziensis , and Zhang et al. [ 107 ] reported four new RNA virus genomic fragments from C. gigas , which were recovered from a virome survey of marine invertebrates. Another 33 novel RNA viruses were identified from mixed bivalve samples (including two oyster species, C. hongkongensis and C. ariakensis ) [ 85 ]. To explore RNA viruses, 33 related libraries (including 19 WTA and 14 RT-WGA libraries) were constructed in this study (Table S 1 ). However, we only recovered 4,958 RNA vOTUs, which accounted for 0.68% of all the viruses in the DOV, and all of them were classified as unknown Riboviria (Fig. S 1 ). Compared with the substantial DNA virus sequence database, the dataset of RNA viruses is exceptionally small. Recently, new approaches were used to optimize the discovery methods of RNA viruses, which has greatly expanded the available RNA virus catalog [ 105 , 62 , 103 ]. We anticipate that more RNA viruses associated with oysters will be explored if these new approaches and the expanded dataset are used.
Ostreid herpesvirus is the most extensively studied DNA viral pathogen for oysters and many other aquaculture bivalves [ 18 , 24 , 28 , 74 , 77 ]. Compared with RNA viruses in the DOV, which have lower diversity, large numbers of DNA viruses were found to have dominated at all the sampling sites (Fig. 1 ), which indicates the importance of DNA viruses in oysters and the marine environment. Consistent with the results of Dupont et al. [ 22 ], viruses in the order Caudovirales dominated the oyster virome (Figs. 2 and S 1 ), just as they dominate the public dataset and culture collections [ 44 ]. The top-three Caudovirales families in the DOV were Siphoviridae (28.5–30.61%), Podoviridae (13.46–42.52%), and Myoviridae (18.36–29.61%) (Fig. 2 A–C). Considering the primary bias of MDA on circular ssDNA genome, Microviridae and Circoviridae accounted for only 2.23% of all the viruses (Fig. S 1 ), which means their diversity may be less than 2.23% and much lower than the diversity of the dsDNA viruses in the DOV.
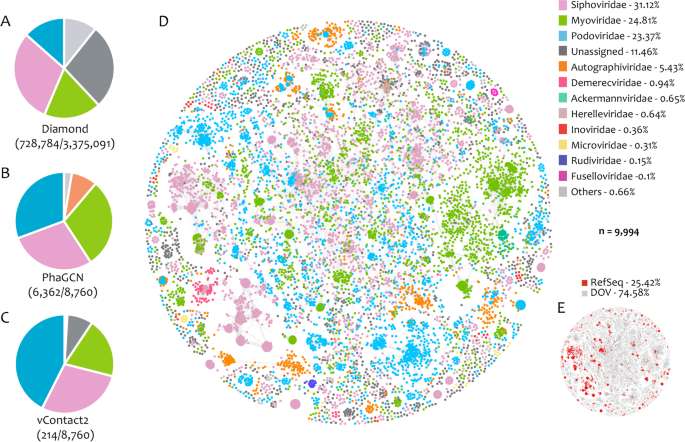
Taxonomy classification of the Dataset of Oyster Virome (DOV) at the family level. A – C Pie charts showing the proportion of different viral families in the total viral contigs (vOTUs) longer than 800 bp. The vOTUs were classified using Diamond v0.9.24.125 ( A ), and vOTUs longer than 10 kb were classified using PhaGCN ( B ) and vContact2 ( C ). The numbers in parentheses indicate the number of vOTU successfully classified/total number of queries. D , E vContact2 networks constructed with vOTUs and NCBI RefSeq viral genomes (release March 2021) longer than 10 kb showing they have the same topology. The colors of the nodes indicate different PhaGCN families ( D ), and their sources ( E ). n , total number of nodes in vContact2 networks. The percentage of each family or source in D and E is listed after the corresponding legends
BLAST-based taxonomy of short contigs has limited accuracy [ 41 ] and a large proportion of them (> 30%) could not be assigned at the family level (Fig. S 1 ). In view of this, PhaGCN was used and successfully classified 6,362 out of 8,760 large vOTUs (of ≥ 10 kb) (Fig. 2 B), which exceeded the number classified by vContact2 (214/8,760) (Fig. 2 C), and the percentage of unassigned vOTUs decreased to 11.46% (Fig. 2 D). Impressively, the DOV nodes (vOTUs) accounted for 74.58% of the total nodes, whereas the RefSeq nodes account for only 25.42% in the vConTACT2 network (Fig. 2 E), indicating that current knowledge about the ocean virosphere is far from sufficient.
Near-complete viral genomes.
A total of 3,473 viral contigs with > 90% genomic completeness (including 27 RNA viral genomes) were identified (Figs. 3 and S 2 ; Table S 2 ). The genome lengths were 1,206–60,277 bp, and the GC content was 24.74–65.70% (Fig. S 2 ). The encoded proteins shared a maximal identity of 0–93.10% (but mainly in the range of 20–40%) with known viral proteins (Fig. 3; Table S 2 ), which again indicated that most of the genomes represented new viral categories. Only 16 of them clustered with nonredundant reference genomes of CheckV, with 95% average nucleotide identity and 70% alignment fraction of contigs. We considered both unknown and unclassified sequences (gray dots in Fig. 3 ) as representing novel viruses at the family level, which account for 67.1% (2,330) of the total (Table S 2 ). The classified genomes belonged to at least 11 DNA virus families; viruses in the order Caudovirales included the Podoviridae (173), Sipoviridae (136), Myoviridae (66), and Autographiviridae (46) (Fig. S 2 ). Circoviridae (order Cirlivirales) and Microviridae (order Petitvirales) were the most abundant families, accounting for 11.27% (396) and 6.98% (240) of the classified genomes, respectively (Fig. 3 ; Table S 2 ).
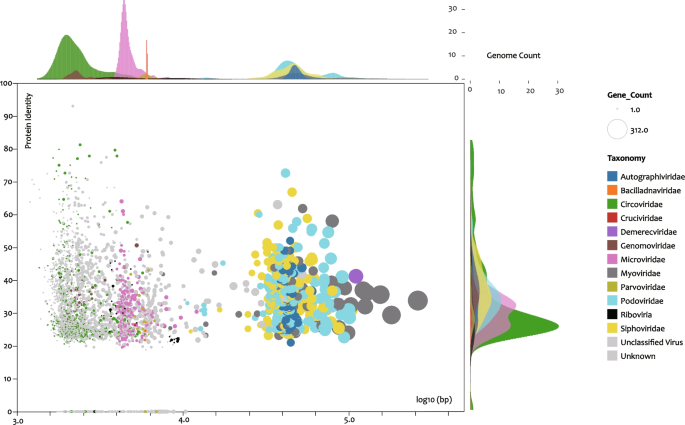
Scatter plot of complete and near-complete viral genomes in the Dataset of Oyster Virome (DOV). X -axis, log10 value of viral genomic length; Y -axis, protein identity between DOV genomes and CheckV reference genomes. The diameter of the circles indicates the gene count in the genomes. Colors indicate different viral families obtained by combining the PhaGCN and Diamond v0.9.24.125 results. The density histograms parallel to the X - and Y -axes show the distribution of the genome count for the corresponding virus families
Among the viruses recognized at the family level, the “Cruciviridae” clade, Genomoviridae, Parvoviridae, and Circoviridae have the potential to infect animals or even humans. The red fire ant is the only known host of members of the Cruciviridae. This species may be related to some small arthropods that are symbiotic or filter-fed in oysters. Viruses in the family Genomoviridae have been recorded to be hosted by a wide range of animals, such as humans [ 98 ], the capybara [ 26 ], tortoises [ 15 ], birds [ 97 ], and many other terrestrial animals. Hosts that have been identified to be infected by members of the Parvoviridae include sea stars [ 39 ], species of Crassostrea [ 43 ] and Fenneropenaeus [ 8 ], seals [ 9 ], humans [ 21 ], and pigeons [ 40 ]. In addition to the well-known circovirus hosts, namely pigs [ 93 ] and birds [ 102 ], circovirus has also been found in several fish species [ 20 , 57 , 58 ], gulls [ 95 ], whales [ 47 ], and humans [ 86 ]. Notably, the discovery of a variety of potential avian viruses reminds us that water contamination from bird feces may be a potential source of marine viruses,therefore, oysters may play an important role as repositories and transmission hotspots of these viruses.
Oyster-related circoviruses
Circovirus was first described in pigs [ 93 ], and together with Cyclovirus, which is found in numerous animal hosts, it forms the family Circoviridae [ 6 ]. Circoviruses are among the smallest animal viruses with an unenveloped icosahedral structure (12–27 nm in diameter), with genomes that mainly include two genes that encode replication initiator protein (Rep) and capsid protein. Circovirus-like genomes have been commonly uncovered in some virome studies, especially investigations employing the MDA method. However, most of the samples analyzed in these studies were environmental or fecal samples [ 14 , 19 , 108 ], which means that it is difficult to determine the exact host of those circovirus-like sequences. As shown from the viral proteomic tree (Fig. S 2 ), circovirus-related branches were widely dispersed and mixed with unannotated branches, implying that many putative circovirus clades are yet to be identified. The fact that all currently known hosts of circoviruses are in clade Bilateria of kingdom Animalia (Virus-Host Database, May 2021: https://www.genome.jp/virushostdb ) suggests that the circoviruses in the DOV were most likely ones hosted by oysters or other multicellular organisms associate with oysters. Although genetic variation in circovirus can occur fast, similar to the properties of some RNA viruses [ 25 ], finding so many circovirus-like genomes in one animal species was quite unexpected.
Furthermore, we used the Rep sequences of circoviruses recorded by the International Committee on Taxonomy of Viruses (ICTV) as queries and mined out 1390 and 8763 nearly complete circovirus-related Rep sequences from the NCBI nr and DOV, respectively, by iterative BlastP searches. Similarity clustering of the identified Rep sequences (Fig. S 3 ) shows that the circovirus-related sequences are very diverse. With the exception of the two Circoviridae genera, Circovirus and Cyclovirus, which have been recorded by the ICTV, most of the other clusters contain sequences that have not been clearly classified (Fig. S 3 ). Among them, the sequences from the DOV accounted for 86.3% (6.3 times the percentage from the NCBI nr) and were widely distributed and present in all the clusters. Some clusters even contained only sequences recorded in the DOV, which indicates that the sequences had not yet been discovered (Fig. S 3 ).
We also constructed a phylogeny (Fig. 4 ) using the Rep sequences that clustered with the circoviruses and cycloviruses (Fig. S 3 ). The results showed that most of the Rep sequences from the DOV were on an independent branch separate from the Circovirus and Cyclovirus branches and distant from the branches of contaminant sequences (excluding the possibility of reagent contamination). We considered that these Rep sequences from the DOV represented a new oyster- or bivalve-specific genus under Circoviridae, and we tentatively named it Crasscircovirus (Fig. 4 ). Five of the DOV sequences were scattered in different Circovirus and Cyclovirus branches (Fig. 4 ). These findings suggest that oysters (and possibly bivalves) may be hotspots of circoviruses. Whether these circoviruses are pathogens or live as symbionts in oyster hosts and whether they will spill over to other marine animals, similar to the behavior of coronavirus in bats, are topics that merit further study [ 96 ].
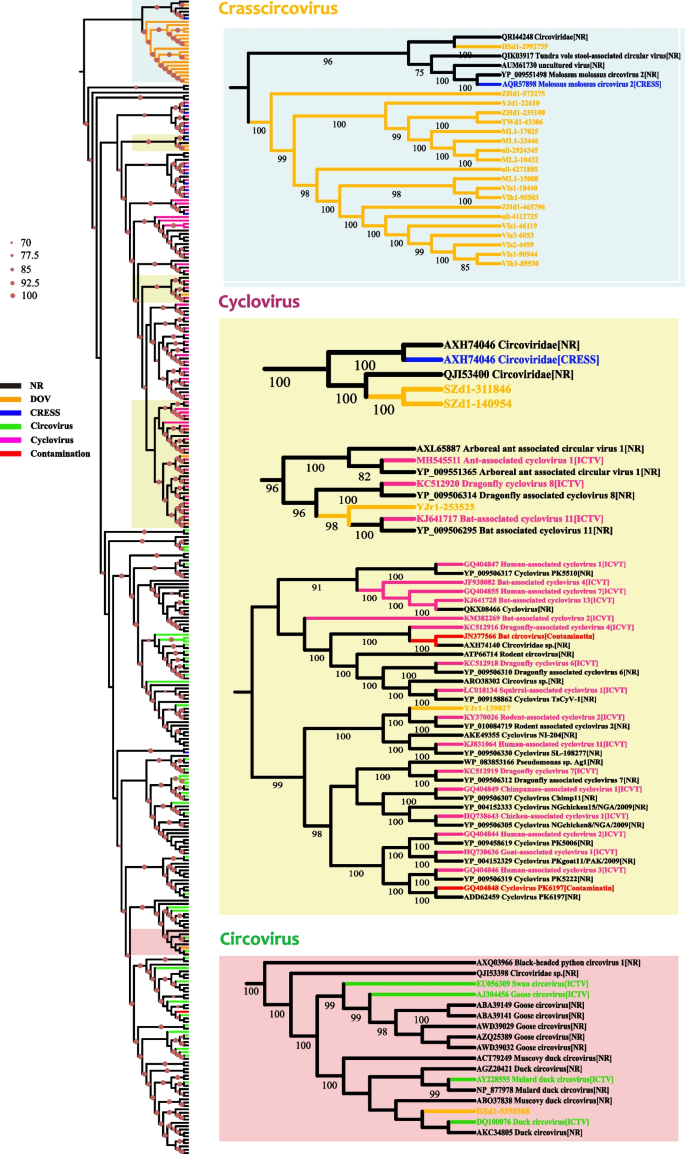
Phylogeny of replication initiator proteins of oyster-related circoviruses. The large tree on the left shows the phylogeny of all proteins in the clusters of the two standard Circoviridae genera recorded by the International Committee on Taxonomy of Viruses (ICTV) (Fig. S 3 ), namely Cyclovirus (green) and Circovirus (violet). The diameter of red dots on branches represents the bootstrap value, and only values above 70 are shown. Small trees on the right are enlarged branches from the tree on the left. Background colors indicate different viral genera: light blue, Crasscircovirus; yellow, Cyclovirus; pink, Circovirus. Colors of branches indicate data origins: orange, Dataset of Oyster Virome (DOV); gray, NCBI nr database; blue, CRESS viruses from Porter et al. [ 72 ], green, circoviruses from the ICTV; violet, cycloviruses from the ICTV; red, contaminant sequences from Asplund et al. [ 3 ] and Porter et al. [ 72 ], black, other NCBI nr sequences
RNA viruses versus DNA viruses
Most previous virome studies focused only on DNA or RNA viruses. Quantitatively comparing the diversity and abundance among RNA and DNA viruses in real environments will likely be very interesting [ 34 , 89 , 109 ]. However, so that we could compare the results, in this study, we used various targeted amplifications to compare DNA and RNA viruses in the same sample separately (WGA and WTA) or simultaneously (RT-WGA).
First, our study shows different amplification strategies can efficiently target different genomes, because the vOTUs of RNA viruses in the WTA libraries significantly outnumber those in the WGA libraries, and vice versa for the DNA viruses (Fig. S 4 A, B). Second, although the differences were not significant, the α-diversity of WGA libraries seems to be higher than WTA libraries (Fig. S 4 D–F), which is consistent with previous observations (Figs. 1 C and S 1 ). It seems to be common that the diversity of DNA viruses in nature and public databases is higher than the diversity of RNA viruses [ 48 , 78 , 79 ]. However, further studies are needed to confirm the conclusion that DNA viruses are more diverse than RNA viruses. Furthermore, the extremely high mutation rates of RNA genomes challenged their detection recall of alignment-based annotations [ 33 , 85 ], and the instability of RNA genomes and potential amplification bias also complicated the comparisons.
Notably, although the diversity of the RNA viruses detected seemed low, their abundance (viral reads ratio) in the WTA libraries was similar to that in WGA libraries and significantly higher than found in RT-WGA libraries (Fig. S 5 A). However, because the samples and tissues used by RT-WGA differed from those used by WTA and WGA, we are unable to determine why the RT-WGA libraries showed a relatively low viral reads ratio. Interestingly, the ratio of Riboviria reach up to 70% (Table S 1 ; Fig. S 5 B), when only a tiny ratio of DNA virus transcripts was detected in some WTA libraries (i.e., ChSZ1604Ra and ChSZ1604Rb) (Fig. S 5 C). The detection of transcripts of DNA viruses in the RNA libraries probably indicates that these DNA viruses are actively replicating in the host cells. However, it does not prove that they are pathogens in oysters, because they could be the viruses of other symbiotic organisms. Nonetheless, the WTA libraries that contained an ultra-high proportion of RNA viruses merit further investigation to determine which kinds of RNA viruses are dominant in the samples and to understand why RNA and DNA viruses seem to utilize different replicating and ecological lifestyles.
Viral communities
MDA introduces bias by prioritizing circular ssDNA genome [ 7 ], and this may have led to the > 80% abundance of circular ssDNA virus in several libraries in this study (Fig. S 5 C). Parras-Moltó et al. [ 68 ] found that ordination plots based on dissimilarities among vOTU profiles showed perfect overlapping of related amplified and unamplified viromes and strong separation from unrelated viromes, which showed that MDA can be used for virus community studies. Studies of virus communities can help determine whether the viruses enriched in oysters can be regarded as an organic whole, similar to viruses in the marine environment, or are simply a random and incidental assembly, as well as whether the community can respond to external influences.
We first evaluated the correlation among various community parameters, including the vOTU counts, the ratio of viral reads, variation in the diversity indexes, and the quantity and quality of sequencing reads (Fig. S 6 ). The α-diversities correlated well among three approaches to deciphering communities (based on the RefSeq, vOTU, and AMG datasets) (Fig. S 6 ), which indicates that the methodologies we used for community analysis verified each other. Second, as we expected, targeted amplification plays a decisive role in the virus community (Fig. 5 A), and this was further verified by our determination of the communities based on reference genomes (Fig. 5 B). Besides the amplification method, the obviously different virus abundance patterns, as revealed by the heatmap (Fig. 5 C) and the F -value ranks (Fig. 5 A), showed prominent differences between tissue groups. Even in a semi-open circulatory system, the virus community in the tissue submerged by hemolymph was quite different from that in the hemolymph itself, which shows that different host tissues had a selective effect on the viruses.
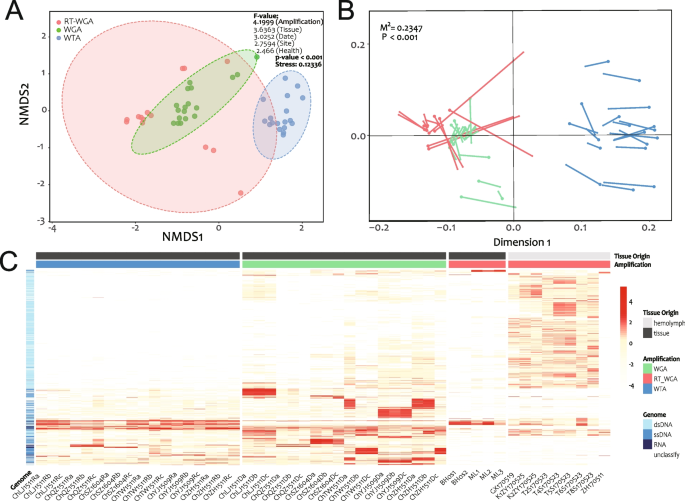
Viral community in the Dataset of Oyster Virome (DOV). A Nonmetric multidimensional scaling (NMDS) analysis shows the clusters of DOV libraries according to amplification groups. Nonparametric multivariate analysis of variance (permanova) was used. RT-WGA, reverse transcription and whole genome amplification; WGA, whole genome amplification; WTA whole transcriptome amplification. B Procrustes analysis of NMDS coordinates of viral communities based on comparisons of reference genomes (RefSeq, GOV, and IMG/VR) and de novo assembled viral contigs (vOTUs). C Heatmap of DOV vOTUs. The vOTUs clustered by the Euclidean method and colored by the viral genome types (dsDNA, ssDNA, RNA, and unclassified) are shown on the Y -axis. The DOV libraries ordered by amplification strategy (WGA, RT_WGA, and WTA) and tissue origin (hemolymph and mixed tissue) are shown on the X -axis
Importantly, although the influence of health status, sampling site, and sampling time on the whole community did not seem to be significant (low F -value) (Fig. 5 A), we still found significant differences in both the α- and β-diversity (NMDS) between all healthy and diseased samples (Fig. S 7 A, C). The α-diversity of moribund groups was relatively high, perhaps signaling that the decrease in immunity caused by disease led to an increase of opportunistic pathogens and their bacteriophages in the host. Dupont et al. [ 22 ] found that the pathogen OsHV-1 μVar virus dominated the hemolymph virome of C. gigas during a disease outbreak, further leading to lower viral diversity than detected in healthy controls. However, the expected differences between moribund and healthy groups were not detected in the parallel cohorts in this study (Fig. S 9 B, C), which suggested that the virus may not be the oyster pathogen.
Geographic origin (sampling site) also substantially influenced the community. Samples from the same location tended to aggregate, and significant differences in α-diversity were observed from the WGA and WTA groups separately (Fig. S 8 ). The influence of the habitat on the microbiome of the host has been reported in many animals [ 29 , 46 , 81 , 90 ] and environmental variations may be one reason for the differences [ 64 ]. However, unlike freely swimming fish, oysters are sedentary and filter large volumes of the surrounding water daily [ 5 , 65 ]. The influence of site on the viromics (viral community) was weaker than that of the time point (lower F -value) (Fig. 5 A), and this was also reflected in the proportion of unique vOTUs (i.e., those detected in only one group) (Fig. S 9 ). The relatively high proportion of unique vOTUs in the time-batch groups implies that viral communities are dynamic with time, and the low proportion of unique vOTUs between sites indicates that viruses were actively exchanged among locations. However, because of the limited sample number, these results need further verification.
Auxiliary metabolic genes (AMGs)
Viruses play essential roles in metabolic regulation in the marine ecosystem [ 10 , 11 , 91 ]. Like marine viruses, a large number (9,091) of AMGs were identified from the DOV. They were assigned to 12 KEGG (Kyoto Encyclopedia of Genes and Genomes) metabolism categories and 98 pathways (Table S 3 ). Among them, pathways associated with the metabolism of cofactors and vitamins, amino acids, energy, and carbohydrates were significantly enriched (Fig. S 10 A), which is similar to the results obtained for other marine viromes [ 16 , 36 , 37 ]. Importantly, the AMG community (Fig. S 10 B) showed consistency with the vOTU community (Fig. S 10 C), and the richness and Shannon index showed positive correlations between the two communities (Figs. S 6 , S 10 D, E). These findings indicate that the oyster viromic function was closely related to that of the species community. Although it is difficult to know which of these is the cause and which is the result, this discovery provides clues that can improve our understanding of the ecological function of the virome in oysters. In addition, the previous finding that viruses with large genomes tend to encode more AMGs than viruses with small genomes, and that they provide ecological functions beyond sustaining basic infection and proliferation [ 42 ], is supported by the results presented in Fig. S 10 F.
Here, we report a comprehensive Dataset of Oyster Virome (DOV) with high resolution, which provides a new resource for studying and understanding the marine virome. Our study describes feasible and targeted protocols for the comparative study of DNA and RNA viromes and suggests that hemolymph may be a suitable tissue for the discovery of viruses in bivalves. Notably, multiple aspects of the research output, including reads recruitment, vOTUs, high-quality virus genomes, and circovirus-related Rep proteins, show that oysters undoubtedly harbor a large, diverse, and unique array of viruses. Oysters may be considered as repositories and transmission hotspots of marine viruses, which is likely an outcome of their filter-feeding lifestyle and the high density of natural populations. In addition, the viral communities in oysters appear to be not random but well organized, and able to respond to changes in host tissues and health state, and in the external environment at both compositional and functional levels. Further studies on the viral community structure and function of bivalves will greatly contribute to the knowledge of their role in coastal microbiome regulation, disease transmission, and potential for protecting and restoring coastal ecosystems.
Availability of data and materials
The data set supporting the results of this article has been deposited in the Genome Sequence Archive and Genome Warehouse in National Genomics Data Center (NGDC) under BioProject accession code PRJCA007058 [ https://ngdc.cncb.ac.cn/bioproject/browse/PRJCA007058 ].
Change history
09 february 2023.
A Correction to this paper has been published: https://doi.org/10.1186/s40168-023-01487-0
Andrade KR, Boratto PP, Rodrigues FP, Silva LC, Dornas FP, Pilotto MR, et al. Oysters as hot spots for mimivirus isolation. Arch Virol. 2015;160(2):477–82.
Article CAS Google Scholar
Apprill A. Marine animal microbiomes: toward understanding host–microbiome interactions in a changing ocean. Front Mar Sci. 2017;4:222.
Article Google Scholar
Asplund M, Kjartansdóttir KR, Mollerup S, Vinner L, Fridholm H, Herrera JA, et al. Contaminating viral sequences in high-throughput sequencing viromics: a linkage study of 700 sequencing libraries. Clin Microbiol Infect. 2019;25(10):1277–85.
Bar-On YM, Phillips R, Milo R. The biomass distribution on Earth. Proc Natl Acad Sci U S A. 2018;115(25):6506–11.
Bedford AJ, Williams G, Bellamy AR. Virus accumulation by the rock oyster Crassostrea glomerata. Appl Environ Microbiol. 1978;35(6):1012–8.
Biagini P, Bendinelli M, Hino S, Kakkola L, Mankertz A, Niel C. Family Circoviridae. In: King AMQ, Adams MJ, Carstens EB, Leftkowitz EJ, editors. Virus taxonomy. IXth Report of the International Committee on Taxonomy of Viruses. London: Elsevier; 2011. 99–123.
Google Scholar
Binga EK, Lasken RS, Neufeld JD. Something from (almost) nothing: the impact of multiple displacement amplification on microbial ecology. ISME J. 2008;2(3):233–41.
Bochow S, Condon K, Elliman J, Owens L. First complete genome of an Ambidensovirus; Cherax quadricarinatus densovirus, from freshwater crayfish Cherax quadricarinatus. Mar Genom. 2015;24:305–12.
Bodewes R, Hapsari R, Rubio Garcia A, Sanchez Contreras GJ, van de Bildt MW, de Graaf M, et al. Molecular epidemiology of seal parvovirus, 1988–2014. PLoS One. 2014;9(11):e112129.
Breitbart M, Bonnain C, Malki K, Sawaya NA. Phage puppet masters of the marine microbial realm. Nat Microbiol. 2018;3(7):754–66. https://doi.org/10.1038/s41564-018-0166-y .
Breitbart M. Marine viruses: truth or dare. Ann Rev Mar Sci. 2012;4:425–48.
Brum JR, Ignacio-Espinoza JC, Roux S, Doulcier G, Acinas SG, Alberti A, Sullivan MB. Patterns and ecological drivers of ocean viral communities. Science. 2015;348(6237):1261498.
Camarillo-Guerrero LF, Almeida A, Rangel-Pineros G, Finn RD, Lawley TD. Massive expansion of human gut bacteriophage diversity. Cell. 2021;184(4):1098-1109.e9.
Castelán-Sánchez HG, Lopéz-Rosas I, García-Suastegui WA, Peralta R, Dobson ADW, Batista-García RA, et al. Extremophile deep-sea viral communities from hydrothermal vents: structural and functional analysis. Mar Genom. 2019;46:16–28.
Chabi-Jesus C, Najar A, Fontenele RS, Kumari SG, Ramos-González PL, Freitas-Astúa J, et al. Viruses representing two new genomovirus species identified in citrus from Tunisia. Arch Virol. 2020;165(5):1225–9.
Castelán-Sánchez HG, Meza-Rodríguez PM, Carrillo E, Ríos-Vázquez DI, Liñan-Torres A, Batista-García RA, et al. The microbial composition in circumneutral thermal springs from Chignahuapan, Puebla, Mexico reveals the presence of particular sulfur-oxidizing bacterial and viral communities. Microorganisms. 2020;8(11):1677. https://doi.org/10.3390/microorganisms8111677 .
Chen S, Zhou Y, Chen Y, Gu J. fastp: an ultrafast all-in-one FASTQ preprocessor. Bioinformatics. 2018;34(17):i884–90.
de Lorgeril J, Lucasson A, Petton B, Toulza E, Montagnani C, Clerissi C, et al. Immune-suppression by OsHV-1 viral infection causes fatal bacteraemia in Pacific oysters. Nat Commun. 2018;9(1):4215.
Dell’Anno A, Corinaldesi C, Danovaro R. Virus decomposition provides an important contribution to benthic deep-sea ecosystem functioning. Proc Natl Acad Sci U S A. 2015;112(16):E2014–9.
CAS Google Scholar
Doszpoly A, Tarján Z, Glávits R, Müller T, Benkő M. Full genome sequence of a novel circo-like virus detected in an adult European eel Anguilla anguilla showing signs of cauliflower disease. Dis Aquat Organ. 2014;109:107–15.
Drexler JF, Reber U, Muth D, Herzog P, Annan A, Ebach F, et al. Human parvovirus 4 in nasal and fecal specimens from children. Ghana Emerg Infect Dis. 2012;18(10):1650–3.
Dupont S, Lokmer A, Corre E, Auguet JC, Petton B, Toulza E. Oyster hemolymph is a complex and dynamic ecosystem hosting bacteria, protists and viruses. Animal Microbiome. 2020;2(1):1–16.
Fan X, Yang C, Li W, Bai X, Zhou X, Xie H, et al. SMOOTH-seq: single-cell genome sequencing of human cells on a third-generation sequencing platform. Genome Biol. 2021;22(1):195.
Farley CA, Banfield WG, Kasnic G Jr, Foster WS. Oyster herpes-type virus. Science. 1972;178(4062):759–60.
Firth C, Charleston MA, Duffy S, Shapiro B, Holmes EC. Insights into the evolutionary history of an emerging livestock pathogen: porcine circovirus 2. J Virol. 2009;83:12813–21.
Fontenele R, Lacorte C, Lamas N, Schmidlin K, Varsani A, Ribeiro S. Single stranded DNA viruses associated with capybara faeces sampled in Brazil. Viruses. 2019;11:710.
Fuhrman JA. Marine viruses and their biogeochemical and ecological effects. Nature. 1999;399:541−8.
Gao F, Jiang JZ, Wang JY, Wei HY. Real-time quantitative isothermal detection of Ostreid herpesvirus-1 DNA in Scapharca subcrenata using recombinase polymerase amplification. J Virol Methods. 2018;255:71–5.
Ge Y, Jing Z, Diao Q, He JZ, Liu YJ. Host species and geography differentiate honeybee gut bacterial communities by changing the relative contribution of community assembly processes. mBio. 2021;12(3):e0075121.
Geoghegan JL, Di Giallonardo F, Wille M, Ortiz-Baez AS, Costa VA, Ghaly T, et al. Virome composition in marine fish revealed by meta-transcriptomics. Virus Evol. 2021;7(1):veab005.
Gregory AC, Zayed AA, Conceição-Neto N, Temperton B, Bolduc B, Alberti A, et al. Marine DNA viral macro- and microdiversity from Pole to Pole. Cell. 2019;177(5):1109–23. https://doi.org/10.1016/j.cell.2019.03.040 .
Holmes EC. Reagent contamination in viromics: all that glitters is not gold. Clin Microbiol Infect. 2019;25(10):1167–8.
Holmes EC. The evolution and emergence of RNA viruses. New York: Oxford Univ Press; 2009.
Holmes EC. What does virus evolution tell us about virus origins? J Virol. 2011;85(11):5247–51.
Hosono S, Faruqi AF, Dean FB, Du Y, Sun Z, Wu X, et al. Unbiased whole-genome amplification directly from clinical samples. Genome Res. 2003;13(5):954–64.
Hurwitz BL, Hallam SJ, Sullivan MB. Metabolic reprogramming by viruses in the sunlit and dark ocean. Genome Biol. 2013;14(11):R123. https://doi.org/10.1186/gb-2013-14-11-r123 .
Hurwitz BL, U’Ren JM. Viral metabolic reprogramming in marine ecosystems. Curr Opin Microbiol. 2016;31:161–8.
Huson DH, Beier S, Flade I, Górska A, El-Hadidi M, Mitra S, et al. MEGAN community edition - interactive exploration and analysis of large-scale microbiome sequencing data. PLoS Comput Biol. 2016;12(6):e1004957.
Jackson EW, Wilhelm RC, Johnson MR, Lutz HL, Danforth I, Gaydos JK, et al. Diversity of sea star-associated densoviruses and transcribed endogenous viral elements of densovirus origin. J Virol. 2020;95(1):e01594–20.
Jager MC, Tomlinson JE, Lopez-Astacio RA, Parrish CR, Van de Walle GR. Small but mighty: old and new parvoviruses of veterinary significance. Virol J. 2021;18(1):210.
Jiang JZ, Zhang W, Guo ZX, Cai CC, Su YL, Wang RX, et al. Functional annotation of an expressed sequence tag library from Haliotis diversicolor and analysis of its plant-like sequences. Mar Genomics. 2011;4(3):189–96.
Jiang T, Guo C, Wang M, Wang M, Zhang X, Liu Y, et al. Genome analysis of two novel Synechococcus phages that lack common auxiliary metabolic genes: possible reasons and ecological insights by comparative analysis of cyanomyoviruses. Viruses. 2020;12(8):800. https://doi.org/10.3390/v12080800 .
Kang YJ, Huang W, Zhao AL, Lai DD, Shao L, Shen YQ, et al. Densoviruses in oyster Crassostrea ariakensis. Arch Virol. 2017;162(7):2153–7.
Kauffman KM, Hussain FA, Yang J, Arevalo P, Brown JM, Chang WK, et al. A major lineage of non-tailed dsDNA viruses as unrecognized killers of marine bacteria. Nature. 2018;554(7690):118–22. https://doi.org/10.1038/nature25474 .
Kieft K, Zhou Z, Anantharaman K. VIBRANT: automated recovery, annotation and curation of microbial viruses, and evaluation of viral community function from genomic sequences. Microbiome. 2020;8(1):90. https://doi.org/10.1186/s40168-020-00867-0 .
Krotman Y, Yergaliyev TM, Alexander Shani R, Avrahami Y, Szitenberg A. Dissecting the factors shaping fish skin microbiomes in a heterogeneous inland water system. Microbiome. 2020;8(1):9.
Landrau-Giovannetti N, Subramaniam K, Brown MA, Ng TFF, Rotstein DS, West K, et al. Genomic characterization of a novel circovirus from a stranded Longman’s beaked whale (Indopacetus pacificus). Virus Res. 2020;277:197826.
Levin RA, Voolstra CR, Weynberg KD, van Oppen MJ. Evidence for a role of viruses in the thermal sensitivity of coral photosymbionts. ISME J. 2017;11(3):808–12. https://doi.org/10.1038/ismej.2016.154 .
Li Y, Fu X, Ma J, Zhang J, Hu Y, Dong W, et al. Altered respiratory virome and serum cytokine profile associated with recurrent respiratory tract infections in children. Nat Commun. 2019;10(1):2288.
Li W, Godzik A. Cd-hit: a fast program for clustering and comparing large sets of protein or nucleotide sequences. Bioinformatics. 2006;22(13):1658–9.
Li D, Liu CM, Luo R, Sadakane K, Lam TW. MEGAHIT: an ultra-fast single-node solution for large and complex metagenomics assembly via succinct de Bruijn graph. Bioinformatics. 2015;31(10):1674–76.
Liu F, Li Y, Yu H, Zhang L, Hu J, Bao Z, et al. MolluscDB: an integrated functional and evolutionary genomics database for the hyper-diverse animal phylum Mollusca. Nucleic Acids Res. 2021;49(D1):D988–97.
Liu P, Chen W, Chen JP. Viral metagenomics revealed Sendai virus and Coronavirus infection of Malayan pangolins (Manis javanica). Viruses. 2019;11(11):979.
Lokmer A, Goedknegt MA, Thieltges DW, Fiorentino D, Kuenzel S, Baines JF, et al. Spatial and temporal dynamics of Pacific oyster hemolymph microbiota across multiple scales. Front Microbiol. 2016;7:1367.
Lokmer A, Kuenzel S, Baines JF, Wegner KM. The role of tissue-specific microbiota in initial establishment success of Pacific oysters. Environ Microbiol. 2016;18(3):970–87.
Lokmer A, Wegner KM. Hemolymph microbiome of Pacific oysters in response to temperature, temperature stress and infection. ISME J. 2015;9(3):670–82.
Lőrincz M, Cságola A, Farkas SL, Székely C, Tuboly T. First detection and analysis of a fish circovirus. J Gen Virol. 2011;192:1817–21.
Lőrincz M, Dán Á, Láng M, Csaba G, Tóth AG, Székely C, et al. Novel circovirus in European catfish (Silurus glanis)[J]. Arch Virol. 2012;157(6):1173–6.
Nayfach S, Camargo AP, Schulz F, Eloe-Fadrosh E, Roux S, Kyrpides NC. CheckV assesses the quality and completeness of metagenome-assembled viral genomes. Nat Biotechnol. 2021;39(5):578–85.
Nayfach S, Páez-Espino D, Call L, Low SJ, Sberro H, Ivanova NN, et al. Metagenomic compendium of 189,680 DNA viruses from the human gut microbiome. Nat Microbiol. 2021;6(7):960–70. https://doi.org/10.1038/s41564-021-00928-6 .
Newell DG, Koopmans M, Verhoef L, Duizer E, Aidara-Kane A, Sprong H, et al. Food-borne diseases - the challenges of 20 years ago still persist while new ones continue to emerge. Int J Food Microbiol. 2010;139(Suppl 1):S3-15.
Neri U, Wolf YI, Roux S, Camargo AP, Lee B, Kazlauskas D, et al. RNA Virus Discovery Consortium, Krupovic M, Dolja VV, Kyrpides NC, Koonin EV, Gophna U. Expansion of the global RNA virome reveals diverse clades of bacteriophages. Cell. 2022 Oct 13;185(21):4023–37.e18.
Nguyen VK, King WL, Siboni N, Mahbub KR, Dove M, O’Connor W, et al. The Sydney rock oyster microbiota is influenced by location, season and genetics. Aquaculture. 2020;527:735472.
Oetama VSP, Hennersdorf P, Abdul-Aziz MA, Mrotzek G, Haryanti H, Saluz HP. Microbiome analysis and detection of pathogenic bacteria of Penaeus monodon from Jakarta Bay and Bali. Mar Pollut Bull. 2016;110(2):718–25.
Olalemi A, Baker-Austin C, Ebdon J, Taylor H. Bioaccumulation and persistence of faecal bacterial and viral indicators in Mytilus edulis and Crassostrea gigas. Int J Hyg Environ Health. 2016;219(7 Pt A):592–8.
Paez-Espino D, Eloe-Fadrosh EA, Pavlopoulos GA, Thomas AD, Huntemann M, Mikhailova N, et al. Uncovering Earth’s virome. Nature. 2016;536(7617):425–30. https://doi.org/10.1038/nature19094 .
Pan X, Durrett RE, Zhu H, Tanaka Y, Li Y, Zi X, et al. Two methods for full-length RNA sequencing for low quantities of cells and single cells. Proc Natl Acad Sci U S A. 2013;110(2):594–9.
Parras-Moltó M, Rodríguez-Galet A, Suárez-Rodríguez P, López-Bueno A. Evaluation of bias induced by viral enrichment and random amplification protocols in metagenomic surveys of saliva DNA viruses. Microbiome. 2018;6(1):119.
Patro R, Duggal G, Love MI, Irizarry RA, Kingsford C. Salmon provides fast and bias-aware quantification of transcript expression. Nat Methods. 2017;14(4):417–9.
Picher ÁJ, Budeus B, Wafzig O, Krüger C, García-Gómez S, Martínez-Jiménez MI, et al. TruePrime is a novel method for whole-genome amplification from single cells based on TthPrimPol. Nat Commun. 2016;7:13296.
Polson SW, Wilhelm SW, Wommack KE. Unraveling the viral tapestry (from inside the capsid out). ISME J. 2011;5(2):165–8.
Porter AF, Cobbin J, Li CX, Eden JS, Holmes EC. Metagenomic identification of viral sequences in laboratory reagents. Viruses. 2021;13(11):2122.
Powell D, Subramanian S, Suwansa-Ard S, Zhao M, O’Connor W, Raftos D, et al. The genome of the oyster Saccostrea offers insight into the environmental resilience of bivalves. DNA Res. 2018;25(6):655–65.
Renault T, Le Deuff RM, Chollet B, Cochennec N, Gérard A. Concomitant herpes-like virus infections in hatchery-reared larvae and nursery-cultured spat Crassostrea gigas and Ostrea edulis. Dis Aquat Organ. 2000;42(3):173–83.
Rosani U, Gerdol M. A bioinformatics approach reveals seven nearly-complete RNA-virus genomes in bivalve RNA-seq data. Virus Res. 2017;239:33–42.
Rosani U, Shapiro M, Venier P, Allam B. A needle in a haystack: tracing bivalve-associated viruses in high-throughput transcriptomic data. Viruses. 2019;11(3):205.
Rosani U, Venier P. Oyster RNA-seq data support the development of Malacoherpesviridae genomics. Front Microbiol. 2017;8:1515.
Rosario R, Fierer N, Miller S, Luongo J, Breitbart M. Diversity of DNA and RNA viruses in indoor air as assessed via metagenomic sequencing. Environ Sci Technol. 2018;52(3):1014–27.
Roux S, Páez-Espino D, Chen IA, Palaniappan K, Ratner A, Chu K, et al. IMG/VR v3: an integrated ecological and evolutionary framework for interrogating genomes of uncultivated viruses. Nucleic Acids Res. 2021;49(D1):D764–75. https://doi.org/10.1093/nar/gkaa946 .
Roux S, Brum JR, Dutilh BE, et al. Ecogenomics and potential biogeochemical impacts of globally abundant ocean viruses. Nature. 2016;537(7622):689-93.
Sandri C, Correa F, Spiezio C, Trevisi P, Luise D, Modesto M, et al. Fecal microbiota characterization of seychelles giant tortoises (Aldabrachelys gigantea) living in both wild and controlled environments. Front Microbiol. 2020;11:569249.
Scanes E, Parker LM, Seymour JR, Siboni N, King WL, Danckert NP, et al. Climate change alters the haemolymph microbiome of oysters. Mar Pollut Bull. 2021;164:111991.
Shang J, Jiang J, Sun Y. Bacteriophage classification for assembled contigs using graph convolutional network. Bioinformatics. 2021;37(Suppl_1):i25–33.
Shi M, Lin XD, Chen X, Tian JH, Chen LJ, Li K, et al. The evolutionary history of vertebrate RNA viruses. Nature. 2018;556(7700):197–202. https://doi.org/10.1038/s41586-018-0012-7 .
Shi M, Lin XD, Tian JH, Chen LJ, Chen X, Li CX, et al. Redefining the invertebrate RNA virosphere. Nature. 2016;540(7634):539–43. https://doi.org/10.1038/nature20167 .
Smits SL, Zijlstra EE, van Hellemond JJ, Schapendonk CM, Bodewes R, Schürch AC, et al. Novel cyclovirus in human cerebrospinal fluid, Malawi, 2010–2011. Emerg Infect Dis. 2013;19(9):1511–3.
Stepanauskas R, Fergusson EA, Brown J, Poulton NJ, Tupper B, Labonté JM, et al. Improved genome recovery and integrated cell-size analyses of individual uncultured microbial cells and viral particles. Nat Commun. 2017;8(1):84.
Stevick RJ, Post AF, Gómez-Chiarri M. Functional plasticity in oyster gut microbiomes along a eutrophication gradient in an urbanized estuary. Animal microbiome. 2021;3(1):1–17.
Steward GF, Culley AI, Mueller JA, Wood-Charlson EM, Belcaid M, Poisson G. Are we missing half of the viruses in the ocean? ISME J. 2013;7(3):672–9. https://doi.org/10.1038/ismej.2012.121 .
Su S, Munganga BP, Du F, Yu J, Li J, Yu F, et al. Relationship between the fatty acid profiles and gut bacterial communities of the Chinese mitten crab (Eriocheir sinensis) from ecologically different habitats. Front Microbiol. 2020;11:565267.
Suttle CA. Viruses in the sea. Nature. 2005;437(7057):356–61.
Suttle CA. Marine viruses - major players in the global ecosystem. Nat Rev Microbiol. 2007;5:801–12.
Tischer I, Gelderblom H, Vetterman W. Koch MAn A very small porcine virus with circular single-stranded DNA. Nature. 1982;295:64–6.
Tithi SS, Aylward FO, Jensen RV, Zhang L. FastViromeExplorer: a pipeline for virus and phage identification and abundance profiling in metagenomics data. PeerJ. 2018;6:e4227.
Todd D, Scott ANJ, Fringuelli E, Shivraprasad HL, Gavier-Widen D, Smyth JA. Molecular characterization of novel circoviruses from finch and gull. Avian Pathol. 2007;36(1):75–81.
Van Brussel K, Holmes EC. Zoonotic disease and virome diversity in bats. Curr Opin Virol. 2021;23(52):192–202.
Varsani A, Krupovic M. Sequence-based taxonomic framework for the classification of uncultured single-stranded DNA viruses of the family Genomoviridae. Virus Evol. 2017;3(1):vew037.
Wang J, Li Y, He X, Ma J, Hong W, Hu F, et al. Gemykibivirus genome in lower respiratory tract of elderly woman with unexplained acute respiratory distress syndrome. Clin Infect Dis. 2019;69(5):861–4.
Wegner KM, Volkenborn N, Peter H, Eiler A. Disturbance induced decoupling between host genetics and composition of the associated microbiome. BMC Microbiol. 2013;13(1):1–12.
Wei HY, Huang S, Wang JY, Gao F, Jiang JZ. Comparison of methods for library construction and short read annotation of shellfish viral metagenomes. Genes Genom. 2018;40(3):281–8.
Wei HY, Huang S, Yao T, Gao F, Jiang JZ, Wang JY. Detection of viruses in abalone tissue using metagenomics technology. Aquac Res. 2018;49(8):2704–13.
Woods LW, Latimer KS, Barr BC, Niagro FD, Campagnoli RP, Nordhausen RW, et al. Circovirus-like infection in a pigeon. J Vet Diagn Invest. 1993;5(4):609–12.
Wolf YI, Silas S, Wang Y, Wu S, Bocek M, Kazlauskas D, et al. Doubling of the known set of RNA viruses by metagenomic analysis of an aquatic virome. Nat Microbiol. 2020 Oct;5(10):1262–70.
Wommack KE, Colwell RR. Virioplankton: viruses in aquatic ecosystems. Microbiol Mol Biol Rev. 2000;64:69−114.
Zayed AA, Wainaina JM, Dominguez-Huerta G, Pelletier E, Guo JR, et al. Cryptic and abundant marine viruses at the evolutionary origins of Earth’s RNA virome. Science. 2022;376(6589):156–62.
Zhang G, Fang X, Guo X, Li L, Luo R, Xu F, et al. The oyster genome reveals stress adaptation and complexity of shell formation. Nature. 2012;490(7418):49–54. https://doi.org/10.1038/nature11413 .
Zhang YY, Chen Y, Wei X, Cui J. Viromes in marine ecosystems reveal remarkable invertebrate RNA virus diversity. Sci China Life Sci. 2021;65(2):426–37. https://doi.org/10.1007/s11427-020-1936-2 .
Zhao L, Rosario K, Breitbart M, Dufy S. Eukaryotic circular rep-encoding single-stranded DNA (CRESS DNA) viruses: ubiquitous viruses with small genomes and a diverse host range. Adv Virus Res. 2019;103:71–133.
Zuo T, Liu Q, Zhang F, Yeoh YK, Wan Y, Zhan H, et al. Temporal landscape of human gut RNA and DNA virome in SARS-CoV-2 infection and severity. Microbiome. 2021;9(1):91.
Download references
Acknowledgements
We thank Dr. Jiang-Yong Wang, Dr. Edward C. Holmes, Dr. Curtis A. Suttle, and Dr. Xu Kevin Zhong for their insightful comments and feedback. We thank Liwen Bianji (Edanz) ( www.liwenbianji.cn/ ) for editing the English text of a draft of this manuscript.
This project was supported by the Key-Area Research and Development Program of Guangdong Province (2022B0202110001) to Jiang JZ; Natural Science Foundation of China (nos. 31972847 and 32172955) to Jiang JZ and Duan M; Financial Fund of the Ministry of Agriculture and Rural Affairs, P. R. of China (NHYYSWZZZYKZX2020) to Zhang DC; the Central Public-Interest Scientific Institution Basal Research Fund, CAFS (nos. 2020TD42 and 2021SD05) to Jiang JZ; the Guangdong Provincial Special Fund for Modern Agriculture Industry Technology Innovation Teams (no. 2019KJ141) to Jiang JZ; and the Earmarked Fund (no. CARS-49) to Ye LT. The funders had no role in the study design, data collection and analysis, decision to publish, or manuscript preparation.
Author information
Yi-Fei Fang
Present address: Shanghai Majorbio Bio-Pharm Technology Co Ltd, Shanghai, 201203, China
Jing-Zhe Jiang and Yi-Fei Fang contributed equally to this work.
Authors and Affiliations
Key Laboratory of South China Sea Fishery Resources Exploitation and Utilization, Ministry of Agriculture and Rural Affairs, South China Sea Fisheries Research Institute, Chinese Academy of Fishery Sciences, Guangzhou, 510300, Guangdong, China
Jing-Zhe Jiang, Tuo Yao, Jie Lu, Ling-Tong Ye & Dian-Chang Zhang
College of Fisheries and Life Science, Shanghai Ocean University, Shanghai, 201306, China
Jing-Zhe Jiang, Yi-Fei Fang, Hong-Ying Wei, Peng Zhu & Min Liu
Guangdong Province Key Laboratory for Biotechnology Drug Candidates, School of Biosciences and Biopharmaceutics, Guangdong Pharmaceutical University, Guangzhou, 510006, Guangdong, China
Jing-Zhe Jiang & Wen-Guang Yuan
Tianjin Agricultural University, Tianjin, 300384, China
Jing-Zhe Jiang, Li-Ling Yang & Ying-Xiang Guo
Guangdong Magigene Biotechnology Co Ltd, Guangzhou, 510000, Guangdong, China
Hong-Ying Wei & Tao Jin
School of Medicine, Sun Yat-Sen University, Shenzhen, 518107, Guangdong, China
Shenzhen Fisheries Development Research Center, Shenzhen, 518067, Guangdong, China
Shao-Kun Shi
Bureau of Agriculture and Rural Affairs of Conghua District, Guangzhou, 510925, Guangdong, China
State Key Laboratory of Freshwater Ecology and Biotechnology, Institute of Hydrobiology, Chinese Academy of Sciences, Wuhan, 430072, China, Hubei
You can also search for this author in PubMed Google Scholar
Contributions
JJZ : conceptualization, methodology, validation, formal analysis, investigation, resources, data curation, visualization, writing—original draft, writing—review and editing, supervision, project administration, and funding acquisition; FYF : validation, formal analysis, investigation, resources, data curation, writing—original draft, and visualization; WHY : formal analysis, investigation, resources, and data curation; ZP , LM , and YWG: formal analysis, investigation, and visualization; YLL and GYX: investigation and resources; JT and SM: writing—review and editing; YT , LJ , SSK , and WM: resources; YLT: resources and funding acquisition; DM and ZDC: resources, supervision, project administration, and funding acquisition. The authors read and approved the final manuscript.
Corresponding authors
Correspondence to Jing-Zhe Jiang , Ming Duan or Dian-Chang Zhang .
Ethics declarations
Ethics approval and consent to participate.
Not applicable.
Consent for publication
Competing interests.
The authors declare that they have no competing interests.
Additional information
Publisher's note.
Springer Nature remains neutral with regard to jurisdictional claims in published maps and institutional affiliations.
The original online version of this article was revised the affiliations for Yi-Fei Fang and Ming Duan were mislabeled. The affiliation 5 of Yi Fei Fang should be "Present address: Shanghai Majorbio Bio-Pharm Technology Co Ltd, Shanghai 201203, China". The affiliation 10 of Ming Duan should be "State Key Laboratory of Freshwater Ecology and Biotechnology, Institute of Hydrobiology, Chinese Academy of Sciences, Wuhan 430072, Hubei, China." The "Present address" at the beginning of affiliation 6 should be also deleted.
Supplementary Information
Additional file 1:, additional file 2:, additional file 3: figure s1..
Doughnut chart of the taxonomy classification of all the viral contigs (vOTUs) in the Dataset of Oyster Virome (DOV). The proportion of different viral families and unclassified vOTUs (≥800 bp) in DOV are based on BLAST searches of the results of Diamond v0.9.24.125 against the NCBI nonredundant protein sequence (nr) database (release March 2021).
Additional file 4: Figure S2.
Viral proteomic phylogenetic tree of complete and near-complete viral genomes in the Dataset of Oyster Virome (DOV). The viral genomes were clustered based on their mutual amino acid identity using ViPTreeGen v1.1.2. The layers from inside to outside show (1) the warning message of CheckV, (2) GC content of the viral genomes, (3) CheckV evaluation methods of genome completeness, (4) log10 value of genomic length, (5) percentage of genome completeness evaluated by CheckV, (6) viral families in order Caudovirales predicted by PhaGCN, and (7) viral families and non-viral annotations of all the genomes obtained by BLAST searches of the results from Diamond v0.9.24.125 against the NCBI nonredundant protein sequence (nr) database (release Mar 2021).
Additional file 5: Figure S3.
Similarity clustering of circovirus-related replicase proteins in Dataset of Oyster Virome (DOV) and NCBI nr. Dots represent different replicase sequences (n=4,716). Edges represent the score value of the Diamond BlastP results; only scores higher than 185.0 are shown. Network clustering was performed using Gephi v0.9.2 under the Fruchterman-Reingold model. Colors of dots indicate different data origins: orange, Dataset of Oyster Virome (DOV); blue, CRESS from Ashleigh et al. (2021); green, circoviruses from the International Committee on Taxonomy of Viruses (ICTV); violet, cycloviruses from the ICTV; red, contaminant sequences from Asplund et al. (2019) and Porter et al. (2021); grey, other NCBI nr sequences.
Additional file 6: Figure S4.
Preference of amplification strategies for the viral community and genome types. (A) Counts of RNA, (B) DNA, and (C) unclassified viral contigs (vOTU) using the WGA and WTA strategies. (D) Richness, (E) Shannon, and (F) Simpson indexes of the three amplification strategies: RT-WGA, reverse transcription and whole genome amplification; WGA, whole genome amplification; WTA whole transcriptome amplification. Different lowercase letters indicate significant differences ( P <0.05; one-way ANOVAs and Tukey-Kramer post hoc comparisons).
Additional file 7: Figure S5.
Actual and relative abundances of virus taxons in the Dataset of Oyster Virome (DOV) libraries. (A) Comparison of the viral reads ratio among three amplification groups; (B) viral reads ratio and (C) relative abundance of the taxons in the 54 DOV libraries (X-axis). Annotations are based on BLAST searches of the results of Diamond v0.9.24.125 against the NCBI nonredundant protein sequence (nr) database (release March 2021). To facilitate the display, the classifications were not unified at the same taxonomic levels.
Additional file 8: Figure S6.
Correlation matrix of oyster viral communities. Red labels (n=10), diversity indexes, viral reads ratio, and vOTU counts based on vOTUs mapping results; black labels (n=7), quality related parameters of library construction and sequencing; blue labels (n=4), diversity indexes and viral ratio based on the reference genomes (RefSeq, GOV, and IMG/VR) mapping results; green labels (n=3): diversity indexes based on the auxiliary metabolic genes (AMGs) mapping results.
Additional file 9: Figure S7.
Influences of health status on the viral community in the Dataset of Oyster Virome (DOV). (A) Nonmetric multidimensional scaling (NMDS) plots of all the libraries (n=54) and (B) the seventh batch (May 2017) (n=9). (C) Comparison α-diversities (Richness, Shannon and Simpson indexes) between healthy and moribund samples corresponding to the NMDS plots in (A) and (B). Blue bar, healthy group; purple bar, moribund group. Different lowercase letters indicate significant differences (P <0.05; one-way ANOVAs and Tukey-Kramer post hoc comparisons).
Additional file 10: Figure S8.
Influences of sampling sites on the viral community in the Dataset of Oyster Virome (DOV). (A, B) Nonmetric multidimensional scaling plots of different sampling sites of the WTA (A) and WGA (B) groups. (C–F) Comparisons of alpha diversity indexes among sampling sites of the WTA (C. D) and WGA (E, F) groups. The colors are used consistently in the figure. Different lowercase letters indicate significant differences (P <0.05; one-way ANOVAs and Tukey-Kramer post hoc comparisons). WGA, whole genome amplification; WTA whole transcriptome amplification.
Additional file 11: Figure S9.
Percentage of unique viral contigs (vOTUs) (detected in only one group) under different grouping methods.
Additional file 12: Figure S10.
Auxiliary metabolic gene (AMG) diversity in the Dataset of Oyster Virome (DOV). (A) Number of detected AMGs assigned to different KEGG metabolic pathways. (B) Nonmetric multidimensional scaling (NMDS) plot of AMG diversity in the DOV libraries (n = 54). (C) Procrustes analysis of NMDS coordinates between the viral contig (vOTU) and AMG communities. The colors are used consistently in (B) and (C): green, WGA; blue. WTA, red. RT-WGA libraries. RT-WGA, reverse transcription and whole genome amplification; WGA, whole genome amplification; WTA whole transcriptome amplification. (D, E) Correlations and linear correlation curves of the Richness (D) and Shannon (E) indexes between vOTUs and AMGs. (F) Correlation between AMG and open reading frame (ORF) counts on the same vOTU.
Additional file 13 Table S1.
Detailed library grouping information and corresponding metadata.
Additional file 14: Table S2.
Near-complete viral genomes in the Dataset of Oyster Virome (DOV) identified by CheckV.
Additional file 15: Table S3.
Counts of auxiliary metabolic genes (AMGs) and corresponding KEGG categories.
Rights and permissions
Open Access This article is licensed under a Creative Commons Attribution 4.0 International License, which permits use, sharing, adaptation, distribution and reproduction in any medium or format, as long as you give appropriate credit to the original author(s) and the source, provide a link to the Creative Commons licence, and indicate if changes were made. The images or other third party material in this article are included in the article's Creative Commons licence, unless indicated otherwise in a credit line to the material. If material is not included in the article's Creative Commons licence and your intended use is not permitted by statutory regulation or exceeds the permitted use, you will need to obtain permission directly from the copyright holder. To view a copy of this licence, visit http://creativecommons.org/licenses/by/4.0/ . The Creative Commons Public Domain Dedication waiver ( http://creativecommons.org/publicdomain/zero/1.0/ ) applies to the data made available in this article, unless otherwise stated in a credit line to the data.
Reprints and permissions
About this article
Cite this article.
Jiang, JZ., Fang, YF., Wei, HY. et al. A remarkably diverse and well-organized virus community in a filter-feeding oyster. Microbiome 11 , 2 (2023). https://doi.org/10.1186/s40168-022-01431-8
Download citation
Received : 09 February 2022
Accepted : 17 November 2022
Published : 07 January 2023
DOI : https://doi.org/10.1186/s40168-022-01431-8
Share this article
Anyone you share the following link with will be able to read this content:
Sorry, a shareable link is not currently available for this article.
Provided by the Springer Nature SharedIt content-sharing initiative
- Crassostrea hongkongensis
- Viral-like particle enrichment
- Mussel Watch
- Multiple displacement amplification
ISSN: 2049-2618
- General enquiries: [email protected]
- Search Menu
- Sign in through your institution
- Featured Articles
- Author Guidelines
- Open Access
- Submission Site
- About Journal of Molluscan Studies
- About the Malacological Society of London
- Editorial Board
- Advertising and Corporate Services
- Journals Career Network
- Self-Archiving Policy
- Dispatch Dates
- Journals on Oxford Academic
- Books on Oxford Academic
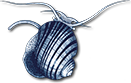
Article Contents
Introduction, material and methods, systematic description, acknowledgements, conflict of interest.
- < Previous
A symbiotic oyster in a shrimp burrow: phylogenetic position of Anomiostrea within the Ostreidae (Bivalvia)

- Article contents
- Figures & tables
- Supplementary Data
Ryutaro Goto, Matteo Garzia, P Graham Oliver, Gustav Paulay, Daniele Salvi, A symbiotic oyster in a shrimp burrow: phylogenetic position of Anomiostrea within the Ostreidae (Bivalvia), Journal of Molluscan Studies , Volume 90, Issue 4, November 2024, eyae034, https://doi.org/10.1093/mollus/eyae034
- Permissions Icon Permissions
The ostreid genus Anomiostrea Habe & Kosuge, 1966, is monotypic for A. coralliophila Habe, 1975, which is known as a symbiont inhabiting the burrow of the ghost shrimp Neocallichirus jousseaumei (Nobili, 1904), but despite this unusual habit among oysters its phylogenetic position within the Ostreidae remained unknown. Using specimens collected from two distant localities of the Indo-Pacific, Oman and Japan, we compared shell morphology of these specimens with the holotype and assessed their phylogenetic relationships based on DNA sequence data from two mitochondrial and two nuclear genes. The genetic distance in cytochrome c oxidase subunit I between the specimens from Japan and Oman was 5.5%, indicating substantial geographic differentiation. Our molecular phylogenetic results suggest that A. coralliophila is sister to Ostrea algoensis G. B. Sowerby II, 1871, an oyster from rocky shores in South Africa, and both are closely related to other Ostrea species. This confirms assignment of Anomiostrea to Ostreinae and suggests that this burrow-wall symbiont evolved from typical rocky-shore oysters. Moreover, A. coralliophila was not monophyletic with another symbiotic ostreid Ostrea permollis G. B. Sowerby II, 1871, nor with other symbiotic oysters, indicating that the symbiotic habit evolved multiple times in the Ostreinae.
The bivalve family Ostreidae Rafinesque, 1815, currently comprises 75 recognized, extant species ( MolluscaBase, 2024 ) including several commercially important oysters. Members of this family inhabit intertidal and shallow subtidal zones worldwide and are assigned to four subfamilies, Crassostreinae Scarlato & Starobogatov, 1979, Ostreinae, Saccostreinae Salvi & Mariottini, 2016, and Striostreinae Harry, 1985 . Two monotypic genera are currently unassigned to any subfamily: Nicaisolopha Vyalov, 1936, and Anomiostrea Habe & Kosuge, 1966 .
Anomiostrea is monotypic for Anomiostrea coralliophila Habe, 1975 . The species name coralliophila is a replacement name for Ostrea pyxidata Adams & Reeve, 1850, a junior homonym of O. pyxidata Born, 1778 ( Habe, 1975 ). The type specimen was collected from the Philippines during the voyage of the “Samarang” ( Adams & Reeve, 1848 ). The species has since been reported across the Indo-West Pacific region, including Kuwait, Sri Lanka, Java, Borneo, the Maluku Islands, the Philippines, and the Ryukyu Islands ( Inaba & Torigoe, 2004 ; Goto, Ohsuga & Kato, 2014 ; GBIF, 2024 ; Oliver et al ., 2023 ). No habitat data were given in the original description of O. pyxidata but Habe & Kosuge (1966 : 338) stated, “found on the corals in the Philippines and rather commonly collected at Samboanga, Mindanao by Mr. Ichiro Yamamura”. The coral association was repeated by Huber (2010 , Chapter 5 CD) “subtidal 2–30 m; coral reefs, cemented on corals and coral branches”. In our study Anomiostrea specimens were found attached to dead coral fragments lining the burrow walls of the ghost shrimp Neocallichirus jousseaumei (Nobili, 1904) as reported by Goto et al. (2014) . Considering the observations in Goto et al. (2014) , it is probable that the specimens in Habe & Kosuge (1966 ) were attached to dead corals that were embedded in the burrow walls of the ghost shrimp, a hypothesis that can be rejected if, in the future, the genus is clearly documented from coral. However, in extensive sampling across the Indo-West Pacific (G. Paulay, personal observations), including numerous collections of coral-associated oysters [ Dendostrea sandvichensis (Sowerby, 1871), Alectryonella plicatula (Gmelin, 1791) and other undescribed and unidentified species], we have never seen Anomiostrea associated with coral.
The symbiotic (i.e. ‘live together’ sensu ; de Bary, 1879 ) association of A. coralliophila with its hosts is likely an example of commensalism. The oyster probably benefits through better protection from predators or environmental stress by living inside the shrimp burrows and may also utilize the water currents created by hosts for filter feeding or respiration ( Goto et al ., 2014 ). The host shrimps may benefit from the existence of A. coralliophila in that the shells of the oyster may stabilize the loose sand or rubble walls of the burrow. This may reduce the time and energy of the host shrimps for burrow maintenance ( Goto et al ., 2014 ). In addition, the host shrimps may gain benefits of better water circulation by A. coralliophila. If the shrimps actually gain some of these benefits from A. coralliophila , their association can be considered one of mutualism.
It is an intriguing question how such a symbiotic habit evolved in the Ostreidae. Several other Ostreinae oysters have symbiotic relationships with other marine invertebrates. Ostrea permollis (Sowerby, 1871) lives embedded in the sponge Stelletta grubii Schmidt, 1862 ( Forbes, 1964 , 1966 ). Alectryonella plicatula (Gmelin, 1791) and two other undescribed/unidentified oysters are obligate associates of living scleractinian corals, while Dendostrea sandvichensis (G. B. Sowerby II, 1871) appears to be a facultative associate (G. Paulay, personal observations). Booneostrea subucula (Jousseaume, 1925) was recorded from the burrows of the axiid shrimp Axiopsis serratifrons (A. Milne-Edwards, 1873) ( Kneer et al ., 2013 ), as similar to A. coralliophila. However, phylogenetic relationships among Ostreinae having a symbiotic habit remain uninvestigated.
Harry (1985) placed Anomiostrea in the Lophinae ( Harry, 1985 ), whereas Huber (2010 ) tentatively placed it in the Ostreinae. Several molecular phylogenetic analyses of the Ostreidae have been conducted ( Foighil & Taylor, 2000 ; Salvi, Macali & Mariottini, 2014 ; Salvi & Mariottini, 2017 , 2021 ; Li et al ., 2021 ), but none included Anomiostrea , thus the phylogenetic position of this lineage remained unclear.
In this work, we studied morphologically identified specimens of A. coralliophila taken from shrimp burrows at two distant localities of the Indo-Pacific—Oman and Japan. We performed the first molecular phylogenetic assessment of Anomiostrea , based on mitochondrial and nuclear markers, to infer its affiliation and also to assess the relationships between Omani and Japanese populations. The main aim of this study is to clarify the systematic position of Anomiostrea and to gain insights on the evolution of the symbiotic habit in oysters.
Sampling and morphological observation
We collected individuals of Anomiostrea coralliophila from the burrows of the ghost shrimp Neocallichirus jousseaumei in Edateku, Amami-Oshima Island, Kagoshima, Japan, in May 2012 ( Goto et al ., 2014 ). At this site, the burrows of N. jousseaumei were often constructed under rocks and thus we turned over rocks embedded in sediments to find the shrimp burrows. In addition, we collected two individuals of A. coralliophila from the burrows of N. jousseaumei in Qurum, Oman. Specimens were levered open and the adductor muscle and mantle excised and fixed in 100% ethanol. Specimens from Japan used for the taxonomic assessment are retained by the first author in the Seto Marine Biological Laboratory, Kyoto University, and those from Oman are deposited in the Florida Museum of Natural History, University of Florida (UF). The holotype of A. coralliophila and specimens from Oman, Japan and Kuwait are illustrated in Fig. 1 . The internal anatomy of a specimen from Japan is illustrated in Fig. 2 .

Anomiostrea coralliophila Habe, 1975 . A–C. Holotype of Ostrea pyxidata Adams & Reeve, 1850. NHMUK 1965114, Philippines. A, B. External and internal surfaces of upper valve. C. Lateral view of external surface of lower valve. D, E. Oman, UF Mollusca 576703. D. External surface of upper valve with juvenile attached. E. Lateral view of whole specimen attached to older upper valve and juvenile attached on top. F–H. Japan, coll, at Seto Marine Biological Laboratory, Kyoto University, not registered. F, G. External and internal surfaces of upper valve with smaller specimen attached. H. Lateral view of external surface of lower valve. I–K. Kuwait, An Niggalayat, ex Smythe coll, NHMUK not registered. I, J. External and internal surfaces of upper valve. K. lateral view of external surface of lower valve.

Internal anatomy of Anomiostrea coralliophila from Japan. Abbreviations: ad, adductor muscle; an, anus; ct, ctenidia; go, gonad; lp, labial palp; re, rectum. Scale bar = 5 mm.
Molecular methods
Genomic DNA was extracted following standard ( Evans, 1990 ) or modified ( Sokolov, 2000 ) high-salt protocols. Two mitochondrial and two nuclear gene fragments were amplified by polymerase chain reaction (PCR): the barcode fragment of cytochrome c oxidase subunit I (COI), and 16S, 18S and 28S ribosomal RNA (rRNA) genes. Amplifications were performed in 25.23 µl mixtures consisting of 0.3 µl forward and reverse primers (10 µM each), 2.5 µl 10xExTaq buffer or reaction buffer (Bioline), 2.0 µl dNTP mixture, 0.13 µl TaKaRa ExTaq polymerase (TaKaRa, Otsu, Japan) or BioTaq (Bioline), 2.5 µl template DNA (at a concentration of ∼20 ng/µl) and 17.5 µl distilled water. The primers used are shown in Table 1 . Thermal cycling was performed with an initial denaturation for 3 min at 94 °C, followed by 30 cycles of 30 s at 94 °C, 30 s at a gene-specific annealing temperature (COI: 42 °C, 16S rRNA, 18S rRNA: 50 °C, 28S rRNA: 50 °C), and 2 min at 72 °C, with a final 3 min extension at 72 °C. PCR products were purified with ExoSAP-IT TM Express PCR Cleanup Reagent (Thermo Fisher Scientific K.K., Tokyo, Japan). The sequencing reaction was performed using the same PCR primers by Eurofins Genomics (Japan) and Genwiz (Germany). Sequences obtained are deposited in the DDBJ/EMBL/GenBank with the accession numbers PP580508 - PP580514 and PP582338 - PP582339 ( Table 2 ).
Primers used in this study.
All primers were used both for the amplification and sequencing except for the 28S rRNA gene for which primers D1 and D3 were used for amplifications and primers D2F and C2R for sequencing.
For the 18S rRNA gene, we produced contigs using fragments sequenced with three primer combinations (1F + 5R, 3F + 18Sbi and 18Sa2.0 + 9R).
GenBank accession numbers for the DNA sequences used for phylogenetic analysis.
Phylogenetic analyses
Chromatograms were edited and assembled using 4Peaks (Griekspoor & Groothuis, nucleobytes.com ) or Geneious v. 11.0.12 (Biomatters Ltd, Auckland, New Zealand). The COI chromatograms did not show any double peak and the translated amino acid sequence did not have any stop codons.
To assess the affiliation of A. coralliophila , we analysed newly generated sequences with homologous sequences of 19 oyster species obtained from GenBank representing all currently accepted genera within the Striostreinae, Saccostreinae and Crassostreinae, six of nine currently accepted genera of Ostreinae ( MolluscaBase, 2024 ), plus two outgroup species from the sister family Gryphaeidae ( Matsumoto, 2003 ; Plazzi et al ., 2011 ; Lemer et al ., 2016 ). Phylogenetic analyses based on this ‘family dataset’ placed A. coralliophila in the Ostreinae. To further assess the relationship of A. coralliophila , we created a second, Ostreinae dataset including 25 species of Ostreinae plus two outgroup species from the Saccostreinae ( Plazzi et al ., 2011 ; Lemer et al ., 2016 ). GenBank accession numbers and references for the DNA sequences used are reported in Table 2 .
Multiple sequence alignments were performed with MAFFT v. 7 on the web server ( Katoh, Rozewicki & Yamada, 2019 ) using the E-INS-i iterative refinement algorithm. Single-gene alignments were concatenated in a single matrix that was used for downstream phylogenetic analyses using maximum likelihood (ML) and Bayesian inference (BI) methods. ML analyses were performed using the IQ-TREE v. 1.6.12 ( Nguyen et al ., 2015 ) based on the best substitution model determined for each gene fragment by the ModelFinder module ( Kalyaanamoorthy et al ., 2017 ) under the corrected Akaike information criterion (cAIC). ML tree searches were based on 20 initial trees until a new best tree could not be found in the last 100 iterations (nstop = 100). Node support was assessed with 1,000 ultrafast bootstrapping pseudoreplicates (uBS). IQ-TREE analyses were performed using the W-IQ-TREE web server ( Trifinopoulos et al ., 2016 ). Bayesian analyses were performed using MrBayes v. 3.2.7 ( Ronquist et al ., 2012 ), using the same substitution models as for the ML analyses. We ran two Markov chains of 5 million generations each, sampled every 500 generations. Consensus trees (50% majority rule) and Bayesian posterior probability values (BPP) were calculated on 7,500 trees sampled after burn-in (25%). Run convergence and effective sample size (ESS) values >200 were assessed using Tracer v. 1.7 ( Rambaut et al ., 2018 ).
Phylogenetic trees were visualized and edited using FigTree v. 1.4.4 ( http://tree.bio.ed.ac.uk/software/figtree/ ). We used MEGA-X v. 10.0.8 ( Tamura, Stecher & Kumar, 2021 ) to compute genetic distances between specimens of Anomiostrea from Oman and Japan based on COI and 16S rRNA sequences.
Molecular phylogenetic analyses
ML and BI analyses based on the family dataset placed Anomiostrea coralliophila within the Ostreinae with strong support (uBS = 98; BPP = 1.0; Fig. 3A ). ML and BI trees had identical topology with four main clades corresponding to the Ostreinae, Crassostreinae, Striostreinae and Saccostreinae, and generic limits matching current systematics of oysters except for the paraphyletic Ostrea and Dendostrea ( Salvi & Mariottini, 2017 ; MolluscaBase, 2024 ).

Phylogenetic tree of Ostreidae ( A ) and Ostreinae ( B ) based on concatenated DNA sequences of COI, 16S rRNA, 28S rRNA and 18S rRNA genes. The topologies of ML and BI trees are identical and only the ML trees are shown. Node support values for ML (ultrafast bootstrapping pseudoreplicates >70) and BI (BPP >0.90) analyses are indicated above and below nodes, respectively.
The phylogenetic trees based on the Ostreinae dataset placed A. coralliophila sister to Ostrea algoensis (uBS = 90; BPP = 1.0; Fig. 3B ), with this lineage sister to a clade comprised of O. futamiensis and O. oleomargarita (uBS = 100; BPP = 1.0), the four species forming a well-supported group (uBS = 83; BPP = 0.98). This clade in turn is sister to a clade that includes the genera Alectryonella, Lopha, Dendostrea, Planostrea plus O. setoensis and O. fluctigera (uBS = 71; BPP = 0.99). This large clade corresponds to the algoensis group described in Salvi et al. (2022) . The average genetic divergence ( p -distance) between specimens of A. coralliophila collected in Oman and Japan is 5.5% for COI and 0.5% for 16S rRNA.
Superfamily OSTREOIDEA Rafinesque, 1815
Family OSTREIDAE Rafinesque, 1815
Subfamily OSTREINAE Rafinesque, 1815
Genus Anomiostrea Habe & Kosuge, 1966
Type species: Anomiostrea coralliophila Habe, 1975 . Replacement name for Ostrea pyxidata Adams & Reeve, 1850.
Original diagnosis of Anomiostrea: “The shell is small, thin, orbicular, dark green to pale yellow, inequivalve. The upper valve is flat, divergently ribbed and has the ovate white muscular scar situated near the centre of its inner surface. The lower valve attached to the coral is deeply concaved, forming a cup-shape and crenulated at the margin by the ribs on the surface and its muscular scar is distinctly elevated by the white callus. The ligament is small and short.”
Diagnosis based on present study : Small oysters to over 35 mm, strongly inequivalve, lower valve deeply cup-shaped, upper valve flat. Juvenile shell more or less smooth, abruptly transitioning to adult sculpture of dense, radial, partly divaricating riblets. Inner margins finely crenulate, aligned with external sculpture. Few sparse, indistinct, ostreine chomata present on dorsolateral margins. Adductor scar raised and thickened toward the posterior.
Remarks : Anomiostrea appears to have been distinguished by the deep, cup-shaped lower valve, flat upper valve, and dense radial, sometimes bifurcating, sculpture. This morphological distinction remains diagnostic, as no other living oysters show such an extreme inequivalve condition.
Anomiostrea coralliophila Habe, 1975
Ostrea pyxidata Adams & Reeve, 1850: 72, pl. 21, fig. 19 (holotype NHMUK 1965114).
Ostrea coralliophila Habe & Kosuge, 1966 : 323, 338, pl. 29, fig. 1.
Anomiostrea coralliophila Habe, 1975 : 184. Inaba & Torigoe, 2004 : 62, pl. 13, figs 2a–d. Huber, 2010 : 179. Goto et al ., 2014 : 201–205, fig. 2.
Material studied : UF Mollusca 576703, Oman, Muscat, Qurum beach and mangroves (23.6249, 58.47861), from Neocallichirus jousseaumei burrows, BOMAN-10623, Lasley, Robert; Paulay, Gustav; Uehling, Abby; Anker, Arthur; Maslakova, Svetlana; Cherneva, Mira; live specimen attached to dead right valve of second specimen. NHMUK, unregistered, Kuwait ex Smythe coll, cited as Ostrea pyxidata in Glayzer, Glayzer & Smythe (1984) , illustrated shells from An Niggalayat. Seto Marine Biological Laboratory, Kyoto University, not registered, Japan, Kagoshima, Edateku, Amami-Oshima Island, intertidal sand flats (28.29, 129.22), from Neocallichirus jousseaumei burrows; live specimen.
Original description of Ostrea pyxidata (translated from the Latin) : Shell 25 mm. Ostr. shell orbicular, inequivalve, left valve flat, radially ribbed, nodular ribs, right convex, radially strongly ribbed, nodular ribs often duplicated, ventral margin crenulated; dirty brown.
Description based on present study : Japanese and Omani specimens (molecularly validated): 21.6 mm (Oman) to 36.3 mm (Japan). Thin, brittle shells. Umbos coiling. Strongly inequivalve. Lower, left valves deeply cupped, depth greatest at the ventral edge, attachment area proportionately large, sides vertical. Upper, right valve more or less flat, umbonal region a little raised. Outline of upper valves subcircular to trigonal, some wider than high, others higher than wide. Sculpture of upper valves initially smooth then abruptly developing radial riblets, increasing through bifurcations to up to 50 at margin, weakly imbricated. Lower valve similar to upper. Inner margin finely crenulate corresponding to external sculpture. Few ostreine chomata on dorsolateral inner margins, most apparent in smaller shells. Adductor scar subcentral, crescentic, becoming swollen and elevated toward posterior ventral edge. Ligament small on a triangular resilifer. Shell colour tan to brown. Juveniles with tendency to attach to adults forming stacks. The gross anatomy is dominated by the large gills with fully reflected paired ctenidia that are attached to the mantle at their distal ends, adductor muscle kidney shaped, mantle has a complex of pallial muscles, labial palps are small, roughly triangular in shape, rectum attached to the posterior of adductor muscle, anus is simple.
Kuwaiti specimens (not molecularly validated): Illustrated shells from An Niggalayat, Kuwait ex Smythe coll, cited as O. pyxidata in Glayzer et al . (1984) . Disarticulated valves only. Small, not exceeding 20 mm in length. Thin, rather brittle. Lower valve deeply cupped, asymmetric with ventral side elevated to 13 mm in 20 mm length, sculptured with fine vertical riblets. Upper valve flat, sculptured by dense narrow riblets, some bifurcating, all slightly imbricated.
Remarks : As would be expected with any oyster species, there is variation in the shell with the outline of the upper valve varying from subcircular to trigonal, lower valve rather shallow (Oman) to almost as deep as long (Kuwait). The expression of the radial riblets also varies. In both Japanese and Oman populations the animals settle upon each other. This may reflect shortage of substrata for attachment but may also provide a reproductive advantage or reflect a direct mode of development.
Anomiostrea coralliophila is recorded across the Indo-West Pacific Oceans from Arabia (Kuwait & Oman) in the west to the Maluku Islands, Philippines, and Ryukyu Islands (Japan) in the east. We sequenced Omani and Japanese specimens, i.e. from these endpoints of its distribution and found them to be 5.5% divergent in COI. This suggests modest allopatric differentiation across the Indo-West Pacific, a common pattern in marine organisms. The level of differentiation found within A. coralliophila is lower than observed in most interspecific comparisons in the Crassostreinae, Saccostreinae and Striostreinae but similar to, or higher than, values observed between closely related Ostreinae such as Ostrea conchaphila / O. lurida, O. angasi / O. edulis, O. equestris / O. stentina or O. puelchana / O. permollis ( Raith et al ., 2015 ; Guo et al ., 2018 ; Hu et al ., 2019 ). Such allopatric differentiation is challenging to interpret in a taxonomic framework as they often represent actively diverging lineages that have not completed the speciation process (see Meyer, Geller & Paulay, 2005 ; Lasley et al ., 2023 ). We thus refrain from making taxonomic decisions on this lineage pending availability and study of more extensive samples.
Our molecular phylogenetic analyses resolved the position of A. coralliophila within the subfamily Ostreinae. The placement of Anomiostrea within Ostreinae was tentatively proposed by Huber (2010 ). Guo et al. (2018) also placed Anomiostrea in the Ostreinae without providing evidence. In this study, beside molecular evidence, the presence of ostreine chomata along the dorsolateral margins of A. coralliophila provides further support for its placement within Ostreinae. Although the soft parts were not fully dissected, the gross anatomy as described above conforms with that of other Ostreinae as described by Torigoe (1981) . There were no apparent modifications of the anatomy that might relate to the small size or unusual habitat of Anomiostrea . Further anatomical comparison between this species and its sister O. algoensis would improve our understanding of this ostreid lineage.
Knowledge on the ecology of A. coralliophila is very limited. Although Habe & Kosuge (1966 ) reported A. coralliophila attached to reef-building corals, the habitat of this species has been clarified by Goto et al. (2014) as a burrow-associated symbiont of the ghost shrimp Neocallichirus jousseaumei . The Omani sample was also taken from the burrows of this ghost shrimp indicating a potentially specific symbiotic relationship. We found A. coralliophila to be sister to O. algoensis described from South Africa where it has been reported to attach to the underside of intertidal rocks ( Kilburn & Rippey, 1982 ; Haupt et al ., 2010 ). Anomiostrea coralliophila is nested within the paraphyletic genus Ostrea ( Fig. 3B ) suggesting that this burrow-symbiont oyster evolved from a group of oysters with a usual lifestyle, sessile on rock surfaces. Our phylogenetic results show that neither O. permollis and A. coralliophila or they with other symbiotic oysters (e.g. Alectryonella plicatula and Dendostrea sandvichensis ) form a monophyletic group ( Fig. 3B ), suggesting a multiple origin of the symbiotic habit in the Ostreinae.
We thank Prof. Makoto Kato and his laboratory members for their support during the field sampling in Edateku, Amami-Oshima Island, Kagoshima, Japan, and Arthur Anker and Rob Lasley for sampling in Oman.
This study was supported by KAKENHI grants to R.G. (numbers 12J07151 and 23K05906). Field work in Oman was supported by NSF DEB 1856245. The work of M.G. at the U.F. malacological collection was supported by the grant ‘Early Career Research Grant—Sir Charles Maurice Yonge Award’ of the Malacological Society of London (2023).
The authors have no conflict of interests to declare.
ADAMS A. , REEVE L.A. 1848 . Mollusca . In: The zoology of the voyage of H.M.S. Samarang, under the command of Captain Sir Edward Belcher, C.B., F.R.A.S., F.G.S., during the years 1843–1846 ( Adams A. ed.). Reeve & Benham , London , x + 87 pp., 24 pls. [Pt. I. Preface to Mollusca (iii–x, by Adams only), 1–24, pls. 1–9, 1 November 1848; Pt. II, 25–44, pls. 10–17, 1 May 1850; Pt. III, Preface and plate explanations (i-xv), 45–87, pls. 18–24, 1 September 1850.] .
Google Scholar
Google Preview
COLGAN D.J. , PONDER W.F. , BEACHAM E. , MACARANAS J.M. 2003 . Gastropod phylogeny based on six segments from four genes representing coding or non-coding and mitochondrial or nuclear DNA . Molluscan Research , 23 : 123 – 148 .
DAYRAT B. , TILLIER A. , LECOINTRE G. , TILLIER S. 2001 . New clades of euthyneuran Gastropods (Mollusca) from 28S rRNA sequences . Molecular Phylogenetics and Evolution , 19 : 225 – 235 .
DE BARY A. 1879 . Die Erscheinung der Symbiose. Vortrag, gehalten auf der Versammlung Deutscher Naturforscher und Aerzte zu Cassel . Trübner Verlag von Karl J. , Strassburg .
EVANS G.A. 1990 . Molecular cloning: A laboratory manual. Second edition. Volumes 1, 2, and 3. Current protocols in molecular biology. Volumes 1 and 2 . Cell , 61 : 17 – 18 .
FOIGHIL D.Ó. , TAYLOR D.J. 2000 . Evolution of parental care and ovulation behavior in oysters . Molecular Phylogenetics and Evolution , 15 : 301 – 313 .
FOLMER O. , BLACK M. , HOEH W. , LUTZ R. , VRIJENHOEK R. 1994 . DNA primers for amplification of mitochondrial cytochrome c oxidase subunit I from diverse metazoan invertebrates . Molecular Marine Biology and Biotechnology , 3 : 294 – 299 .
FORBES M.L. 1964 . Distribution of the commensal oyster, Ostrea permollis , and its host sponge . Bulletin of Marine Science , 14 : 453 – 464 .
FORBES M.L. 1966 . Life cycle of Ostrea permollis and its relationship to the host sponge, Stelletta grubii . Bulletin of Marine Science , 16 : 273 – 301 .
GBIF . 2024 . GBIF occurrence download GBIF.org (05 August 2024). 10.15468/dl.8nuq8d
GIRIBET G. , CARRANZA S. , BAGUÑÀ J. , RIUTORT M. , RIBERA C. 1996 . First molecular evidence for the existence of a Tardigrada + Arthropoda clade . Molecular Biology and Evolution , 13 : 76 – 84 .
GLAYZER B.A. , GLAYZER D.T. , SMYTHE K.R. 1984 . The marine Mollusca of Kuwait, Arabian Gulf . Journal of Conchology , 31 : 311 – 330 .
GOTO R. , OHSUGA K. , KATO M. 2014 . Mode of life of Anomiostrea coralliophila Habe, 1975 (Ostreidae): a symbiotic oyster living in ghost-shrimp burrows . Journal of Molluscan Studies , 80 : 201 – 205 .
GUO X. , LI C. , WANG H. , XU Z. 2018 . Diversity and evolution of living oysters . Journal of Shellfish Research , 37 : 755 – 771 .
HABE T. 1975 . A new name for Anomiostrea pyxidata (Adams & Reeve) (Ostreidae) . Venus , 33 : 184 .
HABE T. , KOSUGE S. 1966 . New genera and species of tropical and subtropical Pacific molluscs . Venus , 24 : 312 – 341 , pl. 29 .
HARRY H.W. 1985 . Synopsis of the supraspecific classification of living oysters (Bivalvia: Gryphaeidae and Ostreidae) . Veliger , 28 : 121 – 158 .
HAUPT T.M. , GRIFFITHS C.L. , ROBINSON T.B. , TONIN A.F.G. , BRUYN P.A.D. 2010 . The history and status of oyster exploitation and culture in South Africa . Journal of Shellfish Research , 29 : 151 – 159 .
HU L. , WANG H. , ZHANG Z. , LI C. , GUO X. 2019 . Classification of small flat oysters of Ostrea stentina species complex and a new species Ostrea neostentina sp. nov. (Bivalvia: Ostreidae) . Journal of Shellfish Research , 38 : 295 – 308 .
HUBER M. 2010 . Compendium of bivalves . Conchbooks , Hackenheim .
INABA A. , TORIGOE K. 2004 . Oysters in the world. Part 2. Systematic description of the recent oysters . Bulletin of the Nishinomiya Shell Museum , 3 : 63 + 9 pp., 13 pls .
KALYAANAMOORTHY S. , MINH B.Q. , WONG T.K.F. , VON HAESELER A. , JERMIIN L.S. 2017 . ModelFinder: fast model selection for accurate phylogenetic estimates . Nature Methods , 14 : 587 – 589 .
KATOH K. , ROZEWICKI J. , YAMADA K.D. 2019 . MAFFT online service: multiple sequence alignment, interactive sequence choice and visualization . Briefings in Bioinformatics , 20 : 1160 – 1166 .
KILBURN R. , RIPPEY E. 1982 . Sea shells of southern Africa . Macmillan , Johannesburg .
KNEER D. , MONINIOT F. , STACH T. , CHRISTIANEN M.J.A . 2013 . Ascidia subterranea sp. nov. (Phlebobranchia: Ascidiidae), a new tunicate belonging to the A. sydneiensis Stimpton, 1855 group, found as burrow associate of Axiopsis serratifrons A. Milne-Edwards, 1873 (Decapoda: Axiidae) on Derawan Island, Indonesia . Zootaxa , 3615 : 485 – 494 .
LASLEY R.M. , EVANS N. , PAULAY G. , MICHONNEAU F. , WINDSOR A. , NG P.K. 2023 . Allopatric mosaics in the Indo-West Pacific crab subfamily Chlorodiellinae reveal correlated patterns of sympatry, genetic divergence, and genitalic disparity . Molecular Phylogenetics and Evolution , 181 : 107710 .
LEMER S. , GONZÁLEZ V.L. , BIELER R. , GIRIBET G . 2016 . Cementing mussels to oysters in the pteriomorphian tree: a phylogenomic approach . Proceedings of the Royal Society B , 283 : 20160857 .
LI C. , KOU Q. , ZHANG Z. , HU L. , HUANG W. , CUI Z. , LIU Y. , MA P. , WANG H. 2021 . Reconstruction of the evolutionary biogeography reveal the origins and diversification of oysters (Bivalvia: Ostreidae) . Molecular Phylogenetics and Evolution , 164 : 107268 .
MATSUMOTO M. 2003 . Phylogenetic analysis of the subclass Pteriomorphia (Bivalvia) from mtDNA COI sequences . Molecular Phylogenetics and Evolution , 27 : 429 – 440 .
MEYER C.P. , GELLER J.B. , PAULAY G. 2005 . Fine scale endemism on coral reefs: archipelagic differentiation in turbinid gastropods . Evolution: International Journal of Organic Evolution , 59 : 113 – 125 .
MOLLUSCABASE . 2024 . Ostreidae Rafinesque, 1815. https://molluscabase.org/aphia.php?p=taxdetails&id=215 (1 January 2024, date last accessed) .
NGUYEN L.-T. , SCHMIDT H.A. , VON HAESELER A. , MINH B.Q. 2015 . IQ-TREE: a fast and effective stochastic algorithm for estimating maximum likelihood phylogenies . Molecular Biology and Evolution , 32 : 268 – 274 .
OLIVER P.G. , AL-KANDARI M.A. , BEHBEHANI M. , DEKKER H. 2023 . An illustrated checklist of the intertidal Bivalvia of the State of Kuwait . Journal of Conchology , 44 : 483 – 528 .
PALUMBI S. , MARTIN A. , ROMANO S. , MCMILLAN W. , STICE L. , GRABOWSKI G. 1991 . The simple fool's guide to PCR . University of Hawaii , Honolulu .
PLAZZI F. , CEREGATO A. , TAVIANI M. , PASSAMONTI M. 2011 . A molecular phylogeny of bivalve mollusks: ancient radiations and divergences as revealed by mitochondrial genes . PLoS ONE , 6 : e27147 .
RAITH M. , ZACHERL D.C. , PILGRIM E.M. , EERNISSE D.J. 2015 . Phylogeny and species diversity of Gulf of California oysters (Ostreidae) inferred from mitochondrial DNA . American Malacological Bulletin , 33 : 1 – 21 .
RAMBAUT A. , DRUMMOND A.J. , XIE D. , BAELE G. , SUCHARD M.A. 2018 . Posterior summarization in Bayesian phylogenetics using Tracer 1.7 . Systematic Biology , 67 : 901 – 904 .
RONQUIST F. , TESLENKO M. , VAN DER MARK P. , AYRES D.L. , DARLING A. , HÖHNA S. , LARGET B. , LIU L. , SUCHARD M.A. , HUELSENBECK J.P . 2012 . MrBayes 3.2: efficient Bayesian phylogenetic inference and model choice across a large model space . Systematic Biology , 61 : 539 – 542 .
SALVI D. , MACALI A. , MARIOTTINI P. 2014 . Molecular phylogenetics and systematics of the bivalve family Ostreidae based on rRNA sequence-structure models and multilocus species tree . PLoS ONE , 9 : e108696 .
SALVI D. , MARIOTTINI P. 2017 . Molecular taxonomy in 2D: A novel ITS2 rRNA sequence-structure approach guides the description of the oysters’ subfamily Saccostreinae and the genus Magallana (Bivalvia: Ostreidae) . Zoological Journal of the Linnean Society , 179 : 263 – 276 .
SALVI D. , MARIOTTINI P. 2021 . Revision shock in Pacific oysters taxonomy: the genus Magallana (formerly Crassostrea in part) is well-founded and necessary . Zoological Journal of the Linnean Society , 192 : 43 – 58 .
SALVI D. , AL-KANDARI M. , OLIVER P.G. , BERRILLI E. , GARZIA M. 2022 . Cryptic marine diversity in the northern Arabian Gulf: an integrative approach uncovers a new species of oyster (Bivalvia: Ostreidae), Ostrea oleomargarita . Journal of Zoological Systematics and Evolutionary Research , 2022 : 7058975 .
SOKOLOV E.P. 2000 . An improved method for DNA isolation from mucopolysaccharide-rich molluscan tissues . Journal of Molluscan Studies , 66 : 573 – 575 .
TAMURA K. , STECHER G. , KUMAR S. 2021 . MEGA11: Molecular Evolutionary Genetics Analysis Version 11 . Molecular Biology and Evolution , 38 : 3022 – 3027 .
TORIGOE K. 1981 . Oysters in Japan . Journal of Science, Hiroshima University, Series B, Division 1 , 29 : 291 – 481 .
TRIFINOPOULOS J. , NGUYEN L.-T. , VON HAESELER A. , MINH B.Q. 2016 . W-IQ-TREE: a fast online phylogenetic tool for maximum likelihood analysis . Nucleic Acids Research , 44 : W232 – W235 .
VONNEMANN V. , SCHRÖDL M. , KLUSSMANN-KOLB A. , WÄGELE H. 2005 . Reconstruction of the phylogeny of the Opisthobranchia (Mollusca: Gastropoda) by means of 18s and 28s rRNA gene sequences . Journal of Molluscan Studies , 71 : 113 – 125 .
Email alerts
Citing articles via.
- X (formerly Twitter)
- Recommend to your Library
Affiliations
- Online ISSN 1464-3766
- Print ISSN 0260-1230
- Copyright © 2024 The Malacological Society of London
- About Oxford Academic
- Publish journals with us
- University press partners
- What we publish
- New features
- Open access
- Institutional account management
- Rights and permissions
- Get help with access
- Accessibility
- Advertising
- Media enquiries
- Oxford University Press
- Oxford Languages
- University of Oxford
Oxford University Press is a department of the University of Oxford. It furthers the University's objective of excellence in research, scholarship, and education by publishing worldwide
- Copyright © 2024 Oxford University Press
- Cookie settings
- Cookie policy
- Privacy policy
- Legal notice
This Feature Is Available To Subscribers Only
Sign In or Create an Account
This PDF is available to Subscribers Only
For full access to this pdf, sign in to an existing account, or purchase an annual subscription.
Academia.edu no longer supports Internet Explorer.
To browse Academia.edu and the wider internet faster and more securely, please take a few seconds to upgrade your browser .
Enter the email address you signed up with and we'll email you a reset link.
- We're Hiring!
- Help Center
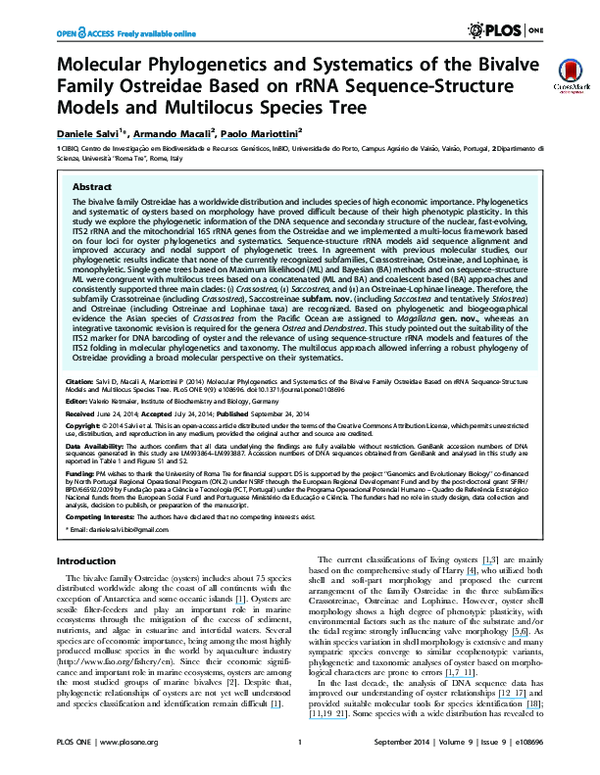
Download Free PDF
Molecular phylogenetics and systematics of the bivalve family Ostreidae based on rRNA sequence-structure models and multilocus species tree

2014, PLoS ONE
Related papers
Zoological Journal of the Linnean Society, 2016
Aquatic Living Resources, 2014
Marine Biology, 2008
Mitochondrial DNA, 2013
The complete mitochondrial (mt) genome of the Olympia oyster Ostrea lurida (16,344 bp), an economically important bivalve, was newly sequenced and annotated. Ostrea lurida is the largest reported Ostrea oyster mt genomes to date and has a comparatively highest overall A + T content (65%) among the available genomes of marine oysters. High levels of variability of nad2 and nad6 genes and that of major non-coding region (MNR) indicate their potential value as useful molecular markers for population and conservation genetic studies in the future. Phylogenetic analyses based on concatenated nucleotide sequences from all 13 PCGs and 2 rRNA genes show that the European flat oyster Ostrea edulis is sister to the Asian slipper oyster Ostrea denselamellosa, while O. lurida is put at the most basal position of the clade, and indicate that Ostrea are closer to Saccostrea than Crassostrea, although gene arrangement shows a closer relationship between Ostrea and Crassostrea. The observations of ...
Conservation Genetics, 2000
The respective status of the Portuguese oyster, Crassostrea angulata, and the Pacific oyster, Crassostrea gigas, has long been a matter of controversy. Morphological and physiological similarities, homogeneity of allozyme allelic frequencies between populations of the two taxa and the demonstration of hybridization lead most authors to suggest that they should be regrouped within the same species. The risk of introgression
Journal of Molluscan Studies, 2007
As a result of phenotypic plasticity, the cupped oysters (Crassostrea) are difficult to identify by means of their morphology. However, molecular DNA markers are a useful means of discriminating among these species. Cupped oysters are one of the most widely cultured marine invertebrates and correct species identification is important in aquaculture. Moreover, the molecular phylogeny of the genus Crassostrea and the subfamily Crassostreinae is still not clear.
Aquaculture, 2004
Molecular Phylogenetics and Evolution, 2010
Zeitschrift für die Alttestamentliche Wissenschaft, 2021
Nature, 2018
Opus supplemento al n.7/2023 Storia architettura restauro disegno / History architecture conservation drawing, 2024
PhD dissertation, University of Manchester, 2019
Journal of Curriculum Studies, 2024
Veleia, 2023
punctum, 2019
Journal of Humanistic Psychology, 1992
Jewish Thought, 2023
International Journal of Advanced Research in Science, Communication and Technology
British Journal of Psychiatry, 1994
South Atlantic Review, 1995
The Baltic Journal of Road and Bridge Engineering, 2016
Journal of Cardiothoracic and Vascular Anesthesia, 2019
Geoforum, 2019
Journal of Pharmaceutical Research International, 2022
International Journal of Engineering Science, 2019
Recycling, 2024
Related topics
- We're Hiring!
- Help Center
- Find new research papers in:
- Health Sciences
- Earth Sciences
- Cognitive Science
- Mathematics
- Computer Science
- Academia ©2024

IMAGES
VIDEO
COMMENTS
Topics Explored: Nitrogen Cycle, Transformation of Energy, Trophic Levels, Food Chains, Food Webs, Population Interactions, Tragedy of the Commons, Ecosystem Services, Eutrophication, Human Impact on Aquatic Ecosystems, AquacultureThis case study explores the unique life cycle of oysters (Family Ostreidae) and their impact on aquatic marine environment, biogeochemical cycles, humans, and the ...
Abstract Oysters (family Ostreidae), with high levels of phenotypic plasticity and wide geographic distribution, are a challenging group for taxonomists and phylogenetics. ... This study used two mitochondrial fragments, cytochrome c oxidase I (COI) and the large ribosomal subunit (16S rDNA), to assess whether oyster species could be identified ...
The bivalve family Ostreidae has a worldwide distribution and includes species of high economic importance. Phylogenetics and systematic of oysters based on morphology have proved difficult because of their high phenotypic plasticity. In this study we explore the phylogenetic information of the DNA sequence and secondary structure of the nuclear, fast-evolving, ITS2 rRNA and the mitochondrial ...
Within the Ostreidae family the results also reveal a closer relationship between Ostrea and Saccostrea than between Ostrea and Crassostrea. ... A case in point is oysters, ... have compared 7 complete mt genomes from Asian oysters. In the present study's aa-based tree built with twelve concatened PCGs from 19 mitochondrial genomes in ...
In the present study we applied, for the first time, an ITS2 rRNA sequence-structure approach for the taxonomic description of a new subfamily and a new genus of oysters, for clades that were uncovered as distinct molecular lineages in a multilocus phylogeny of the bivalve family Ostreidae Rafinesque, 1815 (Salvi et al., 2014).
Phylogeny generated from cytochrome c oxidase I (COI) (short data set) of Ostreidae oysters estimated using neighbour-joining (NJ) approach. Species from family Gryphaeidae are used as outgroup.
The bivalve family Ostreidae (oysters) includes about 75 species distributed worldwide along the coast of all continents with the exception of Antarctica and some oceanic islands . Oysters are sessile filter-feeders and play an important role in marine ecosystems through the mitigation of the excess of sediment, nutrients, and algae in ...
Introduction. Oysters belong to the Phylum Mollusca, Class Bivalvia, Order Pterioida and Family Ostreidae (Zhang and Lou 1956, Ruppert 2013, Bayne et al. 2017).Over 100 species of oysters have been discovered to date (Lindberg and Ponder 2008, Bayne et al. 2017).They have a worldwide distribution and are an important marine biological resource (Bieler et al. 2010, Wu et al. 2011, Salvi et al ...
The bivalve family Ostreidae has a worldwide distribution and includes species of high economic importance. Phylogenetics and systematic of oysters based on morphology have proved difficult ...
Within Ostreidae, molecular analyses revealed new phylogenetic structures that warrant taxonomic consideration. After analyzing 63 oysters with mitochondrial 16S ribosomal RNA (16S) and nuclear 28S ribosomal RNA sequences, Li found high levels of divergence between Crassostrea and Saccostrea and proposed that Saccostrea should be placed into a separate subfamily Saccostreinae Li ().
What family do Oysters belong to? Oysters belong to the family Ostreidae. What order do Oysters belong to? Oysters belong to the order Ostreoida. What type of covering do Oysters have? Oysters are covered in Shells. How many babies do Oysters have? The average number of babies an Oyster has is 1,000,000. What is an interesting fact about Oysters?
Oysters of the family Ostreidae are widely distributed in the intertidal zone globally and are possibly the most highly produced seafood in the world. China is the largest producer of oysters, accounting for 85.3% of the world's total production (FAO, 2019). ... The oyster samples in this study were all adults of C. hongkongensis and the ...
of the bivalve family Ostreidae Rafinesque, 1815 (Salvi et al., 2014). These taxa were given new ... such as oysters provide an excellent case study for molecular taxonomy for two main reasons ...
ABSTRACT. The ostreid genus Anomiostrea Habe & Kosuge, 1966, is monotypic for A. coralliophila Habe, 1975, which is known as a symbiont inhabiting the burrow of the ghost shrimp Neocallichirus jousseaumei (Nobili, 1904), but despite this unusual habit among oysters its phylogenetic position within the Ostreidae remained unknown. Using specimens collected from two distant localities of the Indo ...
Keywords: Oysters, Population structure, Growth patterns 1. Introduction Oyster the member of the family Ostreidae, which are widely consumed by people in the et al. 2013) [18]. Dance (1974)[8] reported that oysters consist of 3 genera, namely Crassostrea, Ostrea, and Lopha. They have an important role as ecosystem formers (Peterson et al. 2003
The family Ostreidae refers to the true oysters. Crassostrea virginica refers to the Virginia Oyster (eastern ... In the case of eastern oysters, no extra filtering system is needed due to filter feeding. ... RP, 1962. Study of oyster growth and population structure of the public reefs in East Bay, Galveston, and Trinity Bay. Study of oyster ...
of the bivalve family Ostreidae Rafinesque, 1815 (Salvi et al., 2014). These taxa were given new ... such as oysters provide an excellent case study for. molecular taxonomy for two main reasons ...
In the present study we applied, for the first time, an ITS2 rRNA sequence-structure approach for the taxonomic description of a new subfamily and a new genus of oysters, for clades that were uncovered as distinct molecular lineages in a multilocus phylogeny of the bivalve family Ostreidae Rafinesque, 1815 (Salvi et al., 2014).
Enter the email address you signed up with and we'll email you a reset link.
The funders had no role in study design, data collection and analysis, decision to publish, or preparation of the manuscript. Competing Interests: The authors have declared that no competing interests exist. * Email: [email protected] Introduction The bivalve family Ostreidae (oysters) includes about 75 species