Thank you for visiting nature.com. You are using a browser version with limited support for CSS. To obtain the best experience, we recommend you use a more up to date browser (or turn off compatibility mode in Internet Explorer). In the meantime, to ensure continued support, we are displaying the site without styles and JavaScript.
- View all journals
- Explore content
- About the journal
- Publish with us
- Sign up for alerts
- Review Article
- Published: 04 March 2009

Animal models of pain: progress and challenges
- Jeffrey S. Mogil 1
Nature Reviews Neuroscience volume 10 , pages 283–294 ( 2009 ) Cite this article
22k Accesses
807 Citations
67 Altmetric
Metrics details
Animal models of pain are crucially important for progress in the field, but the poor translation record of pain research has led to a re-evaluation of the appropriateness of current models.
The accuracy and relevance of data obtained using current animal pain assays can be improved by choosing subjects and conditions that have more in common, epidemiologically and contextually, with pain patients: both sexes, multiple strains, a wider age range, and with attention paid to social factors.
Pain assays have been developed in four successive and overlapping 'waves': acute assays, inflammatory assays, neuropathic assays and the modelling of diseases featuring pain.
Current animal models of pain are overly reliant on innate reflexes as dependent measures. Recently developed operant assays may provide a superior alternative.
Pain researchers seriously under-study spontaneous pain, and are overly reliant on the measurement of hypersensitivity states. A consensus as to which spontaneously emitted behaviours truly reflect pain in laboratory rodents is sorely needed.
Current animal studies of pain largely neglect to measure complex states comorbid with, affected by, and/or contributing to pain.
Many are frustrated with the lack of translational progress in the pain field, in which huge gains in basic science knowledge obtained using animal models have not led to the development of many new clinically effective compounds. A careful re-examination of animal models of pain is therefore warranted. Pain researchers now have at their disposal a much wider range of mutant animals to study, assays that more closely resemble clinical pain states, and dependent measures beyond simple reflexive withdrawal. However, the complexity of the phenomenon of pain has made it difficult to assess the true value of these advances. In addition, pain studies are importantly affected by a wide range of modulatory factors, including sex, genotype and social communication, all of which must be taken into account when using an animal model.
This is a preview of subscription content, access via your institution
Access options
Subscribe to this journal
Receive 12 print issues and online access
176,64 € per year
only 14,72 € per issue
Buy this article
- Purchase on SpringerLink
- Instant access to full article PDF
Prices may be subject to local taxes which are calculated during checkout

Similar content being viewed by others
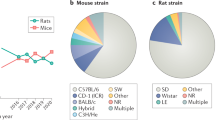
Innovations and advances in modelling and measuring pain in animals

Qualitative sex differences in pain processing: emerging evidence of a biased literature
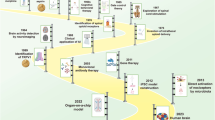
Pathology of pain and its implications for therapeutic interventions
Kola, I. & Landis, J. Can the pharmaceutical industry reduce attrition rates? Nature Rev. Drug Discov. 3 , 711–716 (2004).
Article CAS Google Scholar
Langley, C. K. et al. Volunteer studies in pain research—opportunities and challenges to replace animal experiments: the report and recommendations of a Focus on Alternatives workshop. Neuroimage 42 , 467–473 (2008).
Article CAS PubMed Google Scholar
Hill, R. NK1 (substance P) receptor antagonists–why are they not analgesic in humans? Trends Pharmacol. Sci. 21 , 244–246 (2000). An interesting 'post-mortem' analysis of the failure of NK1 receptor antagonists to show efficacy in humans despite strong preclinical evidence.
Wallace, M. S. et al. A randomized, double-blind, placebo-controlled trial of a glycine antagonist in neuropathic pain. Neurology 59 , 1694–1700 (2002).
Wallace, M. S. et al. A multicenter, double-blind, randomized, placebo-controlled crossover evaluation of a short course of 4030W92 in patients with chronic neuropathic pain. J. Pain 2 , 4227–4233 (2002).
Google Scholar
Williams, J. A., Day, M. & Heavner, J. E. Ziconotide: an update and review. Expert Opin. Pharmacother. 9 , 1575–1583 (2008).
Kontinen, V. K. & Meert, T. F. in Proceedings of the 10th World Congress on Pain (eds Dostrovsky, J. O., Carr, D. B. & Koltzenburg, M.) 489–498 (IASP, Seattle, 2002). The best counterevidence to the charge that animal models of pain fail to predict clinical efficacy; a meta-analysis showing that clinically efficacious treatments for neuropathic pain usually succeed in reversing thermal and mechanical hypersensitivity states in rats.
Whiteside, G. T., Adedoyin, A. & Leventhal, L. Predictive validity of animal pain models? A comparison of the pharmacokinetic-pharmacodynamic relationship for pain drugs in rats and humans. Neuropharmacology 54 , 767–775 (2008).
Campbell, J. N. & Meyer, R. A. Mechanisms of neuropathic pain. Neuron 52 , 77–92 (2006).
Article CAS PubMed PubMed Central Google Scholar
Rice, A. S. C. et al. Animal models and the prediction of efficacy in clinical trials of analgesic drugs: a critical appraisal and call for uniform reporting standards. Pain 139 , 241–245 (2008).
Article Google Scholar
Wilson, S. G. & Mogil, J. S. Measuring pain in the (knockout) mouse: big challenges in a small mammal. Behav. Brain Res. 125 , 65–73 (2001).
Vierck, C. J., Hansson, P. T. & Yezierski, R. P. Clinical and pre-clinical pain assessment: are we measuring the same thing? Pain 135 , 7–10 (2008). A good review of operant measures and their importance.
Mogil, J. S. & Crager, S. E. What should we be measuring in behavioral studies of chronic pain in animals? Pain 112 , 12–15 (2004). A call to include spontaneously emitted behaviours as measures of chronic pain in animals.
Article PubMed Google Scholar
Blackburn-Munro, G. Pain-like behaviours in animals - how human are they? Trends Pharmacol. Sci. 25 , 299–305 (2004).
Stewart, W. F., Ricci, J. A., Chee, E., Morganstein, D. & Lipton, R. Lost productive time and cost due to common pain conditions in the US workforce. J. Am. Med. Assoc. 290 , 2443–2454 (2003).
Price, D. D., McGrath, P., Rafii, A. & Buckingham, B. The validation of visual analogue scales as ratio scale measures for chronic and experimental pain. Pain 17 , 45–56 (1983).
Borsook, D. & Becerra, L. Phenotyping central nervous system circuitry in chronic pain using functional MRI: considerations and potential implications in the clinic. Curr. Pain Headache Rep. 11 , 201–207 (2007).
LaCroix-Fralish, M. L. & Mogil, J. S. Progress in genetic studies of pain and analgesia. Annu. Rev. Pharmacol. Toxicol. 49 , 97–121 (2009). The most current and comprehensive review of pain genetics in animals and humans.
Fields, H. L., Bry, J., Hentall, I. & Zorman, G. The activity of neurons in the rostral medulla of the rat during withdrawal from noxious heat. J. Neurosci. 3 , 2545–2552 (1983).
Mogil, J. S., Simmonds, K. & Simmonds, M. J. Pain research from 1975 to 2007: a categorical and bibliometric meta-trend analysis of every Research Paper published in the journal, Pain . Pain 142 , 48–58 (2009).
Lariviere, W. R., Chesler, E. J. & Mogil, J. S. Transgenic studies of pain and analgesia: mutation or background phenotype? J. Pharmacol. Exp. Ther. 297 , 467–473 (2001).
CAS PubMed Google Scholar
LaCroix-Fralish, M. L., Ledoux, J. B. & Mogil, J. S. The Pain Genes Database : an interactive web browser of pain-related transgenic knockout studies. Pain 131 , 3.e1–4 (2007).
Casas, C. et al. Massive CA1/2 neuronal loss with intraneuronal and N-terminal truncated Aβ42 accumulation in novel Alzheimer transgenic model. Am. J. Pathol. 165 , 1289–1300 (2004).
Indo, Y. et al. Mutations in the TRKA /NGF receptor gene in patients with congenital insensitivity to pain with anhidrosis. Nature Genet. 13 , 485–488 (1996).
Smeyne, R. J. et al. Severe sensory neuropathies in mice carrying a disrupted Trk/NGF receptor gene. Nature 368 , 246–249 (1994).
Nyholt, D. R. et al. A high-density association screen of 155 ion transport genes for involvement with common migraine. Hum. Mol. Genet. 17 , 3318–3331 (2008).
Gagliese, L. & Melzack, R. Chronic pain in elderly people. Pain 70 , 3–14 (1997).
Berkley, K. J. Sex differences in pain. Behav. Brain Sci. 20 , 371–380 (1997). The review paper that first brought the field's attention to the existence of sex differences in pain.
Edwards, R. R., Doleys, D. M., Fillingim, R. B. & Lowery, D. Ethnic differences in pain tolerance: clinical implications in a chronic pain population. Psychosom. Med. 63 , 316–323 (2001).
Mogil, J. S. & Chanda, M. L. The case for the inclusion of female subjects in basic science studies of pain. Pain 117 , 1–5 (2005).
Mogil, J. S., Chesler, E. J., Wilson, S. G., Juraska, J. M. & Sternberg, W. F. Sex differences in thermal nociception and morphine antinociception in rodents depend on genotype. Neurosci. Biobehav. Rev. 24 , 375–389 (2000).
Craft, R. M. Sex differences in drug- and non-drug-induced analgesia. Life Sci. 72 , 2675–2688 (2003).
Kest, B., Wilson, S. G. & Mogil, J. S. Sex differences in supraspinal morphine analgesia are dependent on genotype. J. Pharmacol. Exp. Ther. 289 , 1370–1375 (1999).
Terner, J. M., Lomas, L. M., Smith, E. S., Barrett, A. C. & Picker, M. J. Pharmacogenetic analysis of sex differences in opioid antinociception in rats. Pain 106 , 381–391 (2003).
Mogil, J. S. Sex, gender and pain. Handb. Clin. Neurol. 81 , 325–341 (2006).
Urca, G., Segev, S. & Sarne, Y. Footshock-induced analgesia: its opioid nature depends on the strain of rat. Brain Res. 329 , 109–116 (1985).
Proudfit, H. K. The challenge of defining brainstem pain modulation circuits. J. Pain 3 , 350–354 (2002).
Mogil, J. S. & Belknap, J. K. Sex and genotype determine the selective activation of neurochemically-distinct mechanisms of swim stress-induced analgesia. Pharmacol. Biochem. Behav. 56 , 61–66 (1997).
Le Bars, D., Gozariu, M. & Cadden, S. W. Animal models of nociception. Pharmacol. Rev. 53 , 597–652 (2001). A stunningly comprehensive review of acute and tonic algesiometric assays.
Shir, Y., Ratner, A., Raja, S. N., Campbell, J. N. & Seltzer, Z. Neuropathic pain following partial nerve injury in rats is suppressed by dietary soy. Neurosci. Lett. 240 , 73–76 (1998).
Perez, J., Ware, M. A., Chevalier, S., Gougeon, R. & Shir, Y. Dietary omega-3 fatty acids may be associated with increased neuropathic pain in nerve-injured rats. Anesth. Analg. 101 , 444–448 (2005).
Shir, Y. & Seltzer, Z. Heat hyperalgesia following partial sciatic ligation in rats: interacting nature and nurture. Neuroreport 12 , 809–813 (2001).
Terman, G. W., Shavit, Y., Lewis, J. W., Cannon, J. T. & Liebeskind, J. C. Intrinsic mechanisms of pain inhibition: activation by stress. Science 226 , 1270–1277 (1984).
Imbe, H., Iwai-Liao, Y. & Senba, E. Stress-induced hyperalgesia: animal models and putative mechanisms. Front. Biosci. 11 , 2179–2192 (2006).
Balcombe, J. P., Barnard, N. D. & Sandusky, C. Laboratory routines cause animal stress. Contemp. Top. Lab. Anim. Sci. 43 , 42–51 (2004).
Robinson, I., Dowdall, T. & Meert, T. F. Development of neuropathic pain is affected by bedding texture in two models of peripheral nerve injury in rats. Neurosci. Lett. 368 , 107–111 (2004).
Tjolsen, A. & Hole, K. The tail-flick latency is influenced by skin temperature. APS J. 2 , 107–111 (1993).
Pitcher, G. M., Ritchie, J. & Henry, J. L. Paw withdrawal threshold in the von Frey hair test is influenced by the surface on which the rat stands. J. Neurosci. Methods 87 , 185–193 (1999).
Berman, D. & Rodin, B. E. The influence of housing condition on autotomy following dorsal rhizotomy in rats. Pain 13 , 307–311 (1982).
Raber, P. & Devor, M. Social variables affect phenotype in the neuroma model of neuropathic pain. Pain 97 , 139–150 (2002). An intriguing demonstration of the power of environmental (social) factors to produce effects in a pain assay equal to those of genetic factors.
Callahan, B. L., Gil, A. S. C., Levesque, A. & Mogil, J. S. Modulation of mechanical and thermal nociceptive sensitivity in the laboratory mouse by behavioral state. J. Pain 9 , 174–184 (2008).
Chesler, E. J., Wilson, S. G., Lariviere, W. R., Rodriguez-Zas, S. L. & Mogil, J. S. Identification and ranking of genetic and laboratory environment factors influencing a behavioral trait, thermal nociception, via computational analysis of a large data archive. Neurosci. Biobehav. Rev. 26 , 907–923 (2002).
Langford, D. L. et al. Social modulation of pain as evidence for empathy in mice. Science 312 , 1967–1970 (2006).
Price, D. D. Psychological and neural mechanisms of the affective dimension of pain. Science 288 , 1769–1772 (2000).
Braz, J. M., Nassar, M. A., Wood, J. N. & Basbaum, A. I. Parallel “pain” pathways arise from subpopulations of primary afferent nociceptor. Neuron 47 , 787–793 (2005). This paper suggested that, even in the mouse, the sensory/discriminative component of pain and the motivational/affective component may be dissociable even at the level of primary afferent nociceptor subpopulations.
Hardy, J. D., Wolff, H. G. & Goodell, H. A new method for measuring pain threshold: observations on spatial summation of pain. J. Clin. Invest. 19 , 649–657 (1940).
Mogil, J. S. et al. Screening for pain phenotypes: analysis of three congenic mouse strains on a battery of nine nociceptive assays. Pain 126 , 24–34 (2006).
Dubner, R. in Textbook of Pain (eds Wall, P. D. & Melzack, R.) 247–256 (Churchville Livingstone, Edinburgh, 1989).
Berkley, K. J., Wood, E., Scofield, S. L. & Little, M. Behavioral responses to uterine or vaginal distension in the rat. Pain 61 , 121–131 (1995).
Ness, T. J. & Gebhart, G. F. Colorectal distention as a noxious visceral stimulus: physiologic and pharmacologic characterization of pseudoaffective responses in the rat. Brain Res. 450 , 153–169 (1988).
Hargreaves, K., Dubner, R., Brown, F., Flores, C. & Joris, J. A new and sensitive method for measuring thermal nociception in cutaneous hyperalgesia. Pain 32 , 77–88 (1988).
Koltzenburg, M., Wall, P. D. & McMahon, S. B. Does the right side know what the left is doing? Trends. Neurol. Sci. 22 , 122–127 (1999).
Dubuisson, D. & Dennis, S. G. The formalin test: a quantitative study of the analgesic effects of morphine, meperidine, and brain stem stimulation in rats and cats. Pain 4 , 161–174 (1977).
Dennis, S. G. & Melzack, R. in Advances in Pain Research and Therapy vol. 3 (ed. Bonica, J. J.) 747–760 (Raven, New York, 1979).
Mogil, J. S., Kest, B., Sadowski, B. & Belknap, J. K. Differential genetic mediation of sensitivity to morphine in genetic models of opiate antinociception: influence of nociceptive assay. J. Pharmacol. Exp. Ther. 276 , 532–544 (1996).
McNamara, C. R. et al. TRPA1 mediates formalin-induced pain. Proc. Natl Acad. Sci. USA 104 , 13525–13530 (2007).
Macpherson, L. J. et al. An ion channel essential for sensing chemical damage. J. Neurosci. 27 , 11412–11415 (2007).
Caterina, M. J. et al. The capsaicin receptor: a heat-activated ion channel in the pain pathway. Nature 389 , 816–824 (1997).
Neugebauer, V., Han, J. S., Adwanikar, H., Fu, Y. & Ji, G. Techniques for assessing knee joint pain in arthritis. Mol. Pain 3 , 8 (2007).
Article PubMed PubMed Central Google Scholar
Rang, H. P., Bevan, S. & Dray, A. Chemical activation of nociceptive peripheral neurones. Br. Med. Bull. 47 , 534–548 (1991).
Beecher, H. K. The measurement of pain: prototype for the quantitative study of subjective responses. Pharmacol. Rev. 9 , 59–209 (1957).
Chapman, C. R. et al. Pain measurement: an overview. Pain 22 , 1–31 (1985).
Wall, P. D. et al. Autotomy following peripheral nerve lesions: experimental anaesthesia dolorosa. Pain 7 , 103–113 (1979).
Kauppila, T. Correlation between autotomy-behavior and current theories of neuropathic pain. Neurosci. Biobehav. Rev. 23 , 111–129 (1998).
Bennett, G. J. in Molecular Neurobiology of Pain. Progress in Pain Research and Management vol. 9 (ed. Borsook, D.) 109–113 (IASP, Seattle, 1997).
Bennett, G. J. & Xie, Y.-K. A peripheral mononeuropathy in rat that produces disorders of pain sensation like those seen in man. Pain 33 , 87–107 (1988). The first partial nerve injury animal model; the second most cited research paper ever published in the journal Pain .
Eliav, E., Herzberg, U., Ruda, M. A. & Bennett, G. J. Neuropathic pain from an experimental neuritis of the rat sciatic nerve. Pain 83 , 169–182 (1999).
Chacur, M. et al. A new model of sciatic inflammatory neuritis (SIN): induction of unilateral and bilateral mechanical allodynia following acute unilateral peri-sciatic immune activation in rats. Pain 94 , 231–244 (2001).
DeLeo, J. A. et al. Characterization of a neuropathic pain model: sciatic cryoneurolysis in the rat. Pain 56 , 9–16 (1994).
Gazelius, B., Cui, J.-G., Svensson, M., Meyerson, B. & Linderoth, B. Photochemically induced ischaemic lesion of the rat sciatic nerve. A novel method providing high incidence of mononeuropathy. Neuroreport 7 , 2619–2623 (1996).
Kupers, R., Yu, W., Persson, J. K. E., Xu, X.-J. & Wiesenfeld-Hallin, Z. Photochemically-induced ischemia of the rat sciatic nerve produces a dose-dependent and highly reproducible mechanical, heat and cold allodynia, and signs of spontaneous pain. Pain 76 , 45–59 (1998).
Kim, S. H. & Chung, J. M. An experimental model for peripheral neuropathy produced by segmental spinal nerve ligation in the rat. Pain 50 , 355–363 (1992).
Walczak, J.-S., Pichette, V., Leblond, F., Desbiens, K. & Beaulieu, P. Behavioral, pharmacological and molecular characterization of the saphenous nerve partial ligation: a new model of neuropathic pain. Neuroscience 132 , 1093–1102 (2005).
Mosconi, T. & Kruger, L. Fixed-diameter polyethylene cuffs applied to the rat sciatic nerve induced a painful neuropathy: ultrastructural morphometric analysis of axonal alterations. Pain 64 , 37–57 (1996).
Decosterd, I. & Woolf, C. J. Spared nerve injury: an animal model of persistent peripheral neuropathic pain. Pain 87 , 149–158 (2000).
Seltzer, Z., Dubner, R. & Shir, Y. A novel behavioral model of causalgiform pain produced by partial sciatic nerve injury in rats. Pain 43 , 205–218 (1990).
Djouhri, L., Koutsikou, S., Fang, X., McMullan, S. & Lawson, S. N. Spontaneous pain, both neuropathic and inflammatory, is related to frequency of spontaneous firing in intact C-fiber nociceptors. J. Neurosci. 26 , 1281–1292 (2006).
Kawakami, M. et al. Experimental lumbar radiculopathy, behavior and histologic changes in a model of radicular pain after spinal nerve root irritation with chronic gut ligatures in the rat. Spine 19 , 1795–1802 (1994).
Malmberg, A. B. & Basbaum, A. I. Partial sciatic nerve injury in the mouse as a model of neuropathic pain: behavioral and neuroanatomical correlates. Pain 76 , 215–222 (1998).
Vos, B. P., Strassman, A. M. & Maciewicz, R. J. Behavioral evidence of trigeminal neuropathic pain following chronic constriction injury to the rat's infraorbital nerve. J. Neurosci. 14 , 2708–2723 (1994).
Dowdall, T., Robinson, I. & Meert, T. F. Comparison of five different rat models of peripheral nerve injury. Pharmacol. Biochem. Behav. 80 , 93–108 (2005).
Zeltser, R., Beilin, B.-Z., Zaslansky, R. & Seltzer, Z. Comparison of autotomy behavior induced in rats by various clinically-used neurectomy methods. Pain 89 , 19–24 (2000).
Kim, K. J., Yoon, Y. W. & Chung, J. M. Comparison of three rodent neuropathic models. Exp. Brain Res. 1113 , 200–206 (1997).
Walczak, J.-S. & Beaulieu, P. Comparison of three models of neuropathic pain in mice using a new method to assess cold allodynia: the double plate technique. Neurosci. Lett. 399 , 240–244 (2006).
Cui, J.-G., Holmin, S., Mathiesen, T., Meyerson, B. A. & Linderoth, B. Possible role of inflammatory mediators in tactile hypersensitivity in rat models of mononeuropathy. Pain 88 , 239–248 (2000).
Hall, G. C., Carroll, D., Parry, D. & McQuay, H. J. Epidemiology and treatment of neuropathic pain: the UK primary care perspective. Pain 122 , 156–162 (2006).
Kehlet, H., Jensen, T. S. & Woolf, C. J. Persistent postsurgical pain: risk factors and prevention. Lancet 367 , 1618–1625 (2006).
Nozaki-Taguchi, N. & Yaksh, T. L. A novel model of primary and secondary hyperalgesia after mild thermal injury in the rat. Neurosci. Lett. 254 , 25–28 (1998).
Wang, S. et al. A rat model of unilateral hindpaw burn injury: slowly developing rightwards shift of the morphine dose-response curve. Pain 116 , 87–95 (2005).
Pacharinsak, C. & Beitz, A. Animal models of cancer pain. Comp. Med. 58 , 220–233 (2008). A comprehensive review of an exciting new field of pain research.
CAS PubMed PubMed Central Google Scholar
Coderre, T. J., Xanthos, D. N., Francis, L. & Bennett, G. J. Chronic post-ischemia pain (CPIP): a novel animal model of complex regional pain syndrome-Type I (CRPS-I; reflex sympathetic dystrophy) produced by prolonged hindpaw ischemia and reperfusion in the rat. Pain 112 , 94–105 (2004).
Siegel, S. M., Lee, J. W. & Oaklander, A. L. Needlestick distal nerve injury in rats models symptoms of complex regional pain syndrome. Anesth. Analg. 105 , 1820–1829 (2007).
Bon, K., Lanteri-Minet, M., de Pommery, J., Michiels, J. F. & Menetrey, D. Cyclophosphamide cystitis as a model of visceral pain in rats. A survey of hindbrain structures involved in visceroception and nociception using the expression of c-Fos and Krox-24 proteins. Exp. Brain Res. 108 , 404–416 (1996).
Kuraishi, Y., Nagasawa, T., Hayashi, K. & Satoh, M. Scratching behavior induced by pruritogenic but not algesiogenic agents in mice. Eur. J. Pharmacol. 275 , 229–233 (1995).
Wallace, V. C. J. et al. Characterization of rodent models of HIV-gp120 and anti-retroviral-associated neuropathic pain. Brain 130 , 2688–2702 (2007).
Joseph, E. K., Chen, X., Khasar, S. G. & Levine, J. D. Novel mechanism of enhanced nociception in a model of AIDS therapy-induced painful peripheral neuropathy in the rat. Pain 107 , 147–158 (2004).
Tong, C., Conklin, D. R., Liu, B., Ririe, D. G. & Eisenach, J. C. Assessment of behavior during labor in rats and effect of intrathecal morphine. Anesthesiology 108 , 1081–1086 (2008).
Lynch, J. L., Gallus, N. J., Ericson, M. E. & Beitz, A. J. Analysis of nociception, sex and peripheral nerve innervation in the TMEV animal model of multiple sclerosis. Pain 136 , 293–304 (2008).
Aicher, S. A., Silverman, M. B., Winkler, C. W. & Bebo, B. F. Jr. Hyperalgesia in an animal model of multiple sclerosis. Pain 110 , 560–570 (2004).
Vera-Portocarrero, L. P., Lu, Y. & Westlund, K. N. Nociception in persistent pancreatitis in rats: effects of morphine and neuropeptide alterations. Anesthesiology 98 , 474–484 (2003).
Fleetwood-Walker, S. M. et al. Behavioral changes in the rat following infection with varicella-zoster virus. J. Gen. Virol. 80 , 2433–2436 (1999).
Brennan, T. J., Vandermeulen, E. P. & Gebhart, G. F. Characterization of a rat model of incisional pain. Pain 64 , 493–502 (1996).
Martin, T. J., Buechler, N. L., Kahn, W., Crews, J. C. & Eisenach, J. C. Effects of laparotomy on spontaneous exploratory activity and conditioned operant responding in the rat: a model for postoperative pain. Anesthesiology 101 , 191–203 (2004).
Gonzalez, M. I., Field, M. J., Bramwell, S., McCleary, S. & Singh, L. Ovariohysterectomy in the rat: a model of surgical pain for evaluation of pre-emptive analgesia? Pain 88 , 79–88 (2000).
Wright-Williams, S. L., Courade, J.-P., Richardson, C. A., Roughan, J. V. & Flecknell, P. A. Effects of vasectomy surgery and meloxicam treatment on faecal corticosterone levels and behaviour in two strains of laboratory mouse. Pain 130 , 108–118 (2007).
Rosenzweig, E. S. & McDonald, J. W. Rodent models for treatment of spinal cord injury: research trends and progress toward useful repair. Curr. Opin. Neurol. 17 , 121–131 (2004).
Koo, S. T., Park, Y. I., Lim, K. S., Chung, K. & Chung, J. M. Acupuncture analgesia in a new rat model of ankle sprain pain. Pain 99 , 423–431 (2002).
Fernihough, J. et al. Pain related behaviour in two models of osteoarthritis in the rat knee. Pain 112 , 83–93 (2004).
Bove, S. E. et al. Surgically induced osteoarthritis in the rat results in the development of both osteoarthritis-like joint pain and secondary hyperalgesia. Osteoarthr. Cartil. 14 , 1041–1048 (2006).
Seo, H.-S. et al. A new rat model for thrombus-induced ischemic pain (TIIP); development of bilateral mechanical allodynia. Pain 139 , 520–532 (2008).
Giamberardino, M. A., Valente, R., de Bigontina, P. & Vecchiet, L. Artificial ureteral calculosis in rats: behavioural characterization of visceral pain episodes and their relationship with referred lumbar muscle hyperalgesia. Pain 61 , 459–469 (1995).
Courteix, C., Eschalier, A. & Lavarenne, J. Streptozotocin-induced diabetic rats: behavioural evidence for a model of chronic pain. Pain 53 , 81–88 (1993).
Tesch, G. H. & Allen, T. J. Rodent models of streptozotocin-induced diabetic nephropathy. Nephrology 12 , 261–266 (2007).
Sullivan, K. A., Lentz, S. I., Roberts, J. L. Jr. & Feldman, E. L. Criteria for creating and assessing mouse models of diabetic neuropathy. Curr. Drug Targets 9 , 3–13 (2008).
Fox, A., Eastwood, C., Gentry, C., Manning, D. & Urban, L. Critical evaluation of the streptozotocin model of painful diabetic neuropathy in the rat. Pain 81 , 307–316 (1999).
Hashizume, H., DeLeo, J. A., Colburn, R. W. & Weinstein, J. N. Spinal glial activation and cytokine expression following lumbar root injury in the rat. Spine 25 , 1206–1217 (2000).
Hu, S.-J. & Xing, J.-L. An experimental model for chronic compression of dorsal root ganglion produced by intervertebral foramen stenosis in the rat. Pain 77 , 15–23 (1998).
Olmarker, K., Iwabuchi, M., Larsson, K. & Rydevik, B. Walking analysis of rats subjected to experimental disc herniation. Eur. Spine J. 7 , 394–399 (1998).
CAS Google Scholar
Olmarker, K. Puncture of a lumbar intervertebral disc induces changes in spontaneous pain behavior: an experimental study in rats. Spine 33 , 850–855 (2008).
Kawakami, M. et al. Pathomechanism of pain-related behavior produced by allografts of intervertebral disc in the rat. Spine 21 , 2101–2107 (1996).
Cuellar, J. M., Montesano, P. X. & Carstens, E. Role of TNF-alpha in sensitization of nociceptive dorsal horn neurons induced by application of nucleus pulposus to L5 dorsal root ganglion in rats. Pain 110 , 578–587 (2004).
Kauppila, T., Kontinen, V. K. & Pertovaara, A. Influence of spinalization on spinal withdrawal reflex responses varies depending on the submodality of the test stimulus and the experimental pathophysiological condition in the rat. Brain Res. 797 , 234–242 (1998).
Franklin, K. B. J. & Abbott, F. V. in Neuromethods, Vol. 13: Psychopharmacology (eds Boulton, A. A., Baker, G. B. & Greenshaw, A. J.) (Humana, Clifton, New Jersey, 1989).
Matthies, B. K. & Franklin, K. B. J. Formalin pain is expressed in decerebrate rats but not attenuated by morphine. Pain 51 , 199–206 (1992).
Woolf, C. J. Long term alterations in excitability of the flexion reflex produced by peripheral tissue injury in the chronic decerebrate rat. Pain 18 , 325–343 (1984).
Guilbaud, G. et al. Time course of degeneration and regeneration of myelinated nerve fibres following chronic loose ligatures of the rat sciatic nerve: can nerve lesions be linked to the abnormal pain-related behaviours. Pain 53 , 147–158 (1993).
Kauppila, T., Kontinen, V. K. & Pertovaara, A. Weight bearing of the limb as a confounding factor in assessment of mechanical allodynia in the rat. Pain 74 , 55–59 (1998).
Backonja, M.-M. & Stacey, B. Neuropathic pain symptoms relative to overall pain rating. J. Pain 5 , 491–497 (2004). A survey of the clinical symptoms of neuropathic pain patients. It revealed that spontaneous pain is more prevalent, and more reflective of overall pain ratings, than mechanical and thermal hypersensitivities.
Gottrup, H., Nielsen, J., Arendt-Nielsen, L. & Jensen, T. S. The relationship between sensory thresholds and mechanical hyperalgesia in nerve injury. Pain 75 , 321–329 (1998).
Rowbotham, M. C. & Fields, H. L. The relationship of pain, allodynia and thermal sensation in post-herpetic neuralgia. Brain 119 , 347–354 (1996).
Kupers, R. C., Nuytten, D., De Castro-Costa, M. & Gybels, J. M. A time course analysis of the changes in spontaneous and evoked behaviour in a rat model of neuropathic pain. Pain 50 , 101–111 (1992).
Meller, S. T. Thermal and mechanical hyperalgesia: a distinct role for different excitatory amino acid receptors and signal transduction pathways? APS J. 3 , 215–231 (1994).
Mogil, J. S. et al. Heritability of nociception. II. “Types” of nociception revealed by genetic correlation analysis. Pain 80 , 83–93 (1999).
Hansson, P. Difficulties in stratifying neuropathic pain by mechanisms. Eur. J. Pain 7 , 353–357 (2003).
Field, M. J., Bramwell, S., Hughes, J. & Singh, L. Detection of static and dynamic components of mechanical allodynia in rat models of neuropathic pain: are they signalled by distinct primary sensory neurones? Pain 83 , 303–311 (1999).
Loeser, J. D. Pain and suffering. Clin. J. Pain 16 (Suppl. 2), S2–S6 (2000).
Warner, L. H. The association span of the white rat. J. Genet. Psychol. 41 , 57–89 (1932).
Weiss, B. & Laties, V. G. Fractional escape and avoidance on a titration schedule. Science 128 , 1575–1576 (1958).
Bodnar, R. J., Kelly, D. D., Brutus, M., Mansour, A. & Glusman, M. 2-Deoxy-D-glucose-induced decrements in operant and reflex pain thresholds. Pharmacol. Biochem. Behav. 9 , 543–549 (1978).
Ross, E. L., Komisaruk, B. R. & O'Donnell, D. Evidence that probing the vaginal cervix is analgesic in rats, using an operant paradigm. J. Comp. Physiol. Psychol. 93 , 330–336 (1979).
Mauderli, A. P., Acosta-Rua, A. & Vierck, C. J. An operant assay of thermal pain in conscious, unrestrained rats. J. Neurosci. Methods 97 , 19–29 (2000).
Baliki, M., Calvo, O., Chialvo, D. R. & Apkarian, A. V. Spared nerve injury rats exhibit thermal hyperalgesia on an automated operant dynamic thermal escape task. Mol. Pain 1 , 18 (2005).
PubMed PubMed Central Google Scholar
Ding, H. K., Shum, F. W., Ko, S. W. & Zhuo, M. A new assay of thermal-based avoidance test in freely moving mice. J. Pain 6 , 411–416 (2005).
Dubner, R., Beitel, R. E. & Brown, F. J. in Pain: New Perspectives in Therapy and Research (eds Weisenberg, M. & Tursky, B.) 155–170 (Plenum, New York, 1976).
Book Google Scholar
Sufka, K. J., Brockel, B. J., Liou, J.-R. & Fowler, S. C. Functional deficits following bilateral forelimb adjuvant inflammation assessed by operant methodology: effects of indomethacin and morphine on recovery of function. Exp. Clin. Psychopharmacol. 4 , 336–343 (1996).
Neubert, J. K. et al. Use of a novel thermal operant behavioral assay for characterization of orofacial pain sensitivity. Pain 116 , 386–395 (2005).
Thut, P. D., Hermanstyne, T. O., Flake, N. M. & Gold, M. S. An operant conditioning model to assess changes in feeding behavior associated with temporomandibular joint inflammation in the rat. J. Orofac. Pain 21 , 7–18 (2007).
PubMed Google Scholar
Martin, T. J. & Ewan, E. Chronic pain alters drug self-administration: implications for addiction and pain mechanisms. Exp. Clin. Psychopharmacol. 16 , 357–366 (2008).
Sufka, K. J. Conditioned place preference paradigm: a novel approach for analgesic drug assessment against chronic pain. Pain 58 , 355–366 (1994).
Johansen, J. P., Fields, H. L. & Manning, B. H. The affective component of pain in rodents: direct evidence for a contribution of the anterior cingulate cortex. Proc. Natl Acad. Sci. USA 98 , 8077–8082 (2001).
LaBuda, C. J. & Fuchs, P. N. A behavioral test paradigm to measure the aversive quality of inflammatory and neuropathic pain in rats. Exp. Neurol. 163 , 490–494 (2000).
Colpaert, F. C. et al. Opiate self-administration as a measure of chronic nociceptive pain in arthritic rats. Pain 92 , 33–45 (2001).
Neubert, J. K., Rossi, H. L., Malphurs, W., Vierck, C. J. Jr. & Caudle, R. M. Differentiation between capsaicin-induced allodynia and hyperalgesia using a thermal operant assay. Behav. Brain Res. 170 , 308–315 (2006).
Morton, D. B. & Griffiths, P. H. Guidelines on the recognition of pain, distress and discomfort in experimental animals and an hypothesis for assessment. Vet. Rec. 116 , 431–436 (1985).
Stasiak, K. L., Maul, D., French, E., Hellyer, P. W. & Vandewoude, S. Species-specific assessment of pain in laboratory animals. Contemp. Top. Lab. Anim. Sci. 42 , 13–20 (2003).
Williams, W. O., Riskin, D. K. & Mott, K. M. Ultrasonic sound as an indicator of acute pain in laboratory mice. J. Am. Assoc. Lab. Anim. Sci. 47 , 8–10 (2008).
Jourdan, D., Ardid, D. & Eschalier, A. Analysis of ultrasonic vocalisation does not allow chronic pain to be evaluated in rats. Pain 95 , 165–173 (2002).
Han, J. S., Bird, G. C., Li, W., Jones, J. & Neugebauer, V. Computerized analysis of audible and ultrasonic vocalizations of rats as a standardized measure of pain-related behavior. J. Neurosci. Methods 141 , 261–269 (2005).
Calvino, B., Besson, J. M., Boehrer, A. & Depaulis, A. Ultrasonic vocalization (22–28 kHz) in a model of chronic pain, the arthritic rat: effects of analgesic drugs. Neuroreport 7 , 581–584 (1996).
Wallace, V. C. J., Norbury, T. A. & Rice, A. S. C. Ultrasound vocalisation by rodents does not correlate with behavioural measures of persistent pain. Eur. J. Pain 9 , 445–452 (2005).
Roughan, J. V. & Flecknell, P. A. Evaluation of a short duration behaviour-based post-operative pain scoring system in rats. Eur. J. Pain 7 , 397–406 (2003).
Tjolsen, A., Berge, O.-G., Hunskaar, S., Rosland, J. H. & Hole, K. The formalin test: an evaluation of the method. Pain 51 , 5–17 (1992).
Mogil, J. S., Lichtensteiger, C. A. & Wilson, S. G. The effect of genotype on sensitivity to inflammatory nociception: characterization of resistant (A/J) and sensitive (C57BL/6) inbred mouse strains. Pain 76 , 115–125 (1998).
Gould, H. J. Complete Freund's adjuvant-induced hyperalgesia: a human perception. Pain 85 , 301–303 (2000). An intriguing and amusing first-person recounting of symptoms following accidental injection of the inflammatory compound.
Olmarker, K., Storkson, R. & Berge, O.-G. Pathogenesis of sciatic pain: a study of spontaneous behavior in rats exposed to experimental disc herniation. Spine 27 , 1312–1317 (2002).
Roughan, J. V. & Flecknell, P. A. Behavioural effects of laparotomy and analgesic effects of ketoprofen and carprofen in rats. Pain 90 , 65–74 (2001).
Na, H. S., Yoon, Y. W. & Chung, J. M. Both motor and sensory abnormalities contribute to changes in foot posture in an experimental rat neuropathic model. Pain 67 , 173–178 (1996).
van Loo, P. L. P. et al. Analgesics in mice used in cancer research: reduction of discomfort? Lab. Anim. 31 , 318–325 (1997).
D'Almeida, J. A. C. et al. Behavioral changes of Wistar rats with experimentally-induced painful diabetic neuropathy. Arq. Neuropsiquiatr. 57 , 746–752 (1999).
Loeser, J. D., Butler, S. H., Chapman, C. R. & Turk, D. C. (eds) Bonica's Management of Pain 3rd edn (Lippincott Williams & Wilkins, New York, 2000).
Hummel, M., Lu, P., Cummons, T. A. & Whiteside, G. T. The persistence of a long-term negative affective state following the induction of either acute or chronic pain. Pain 140 , 436–445 (2008).
Benbouzid, M. et al. Sciatic nerve cuffing in mice: a model of sustained neuropathic pain. Eur. J. Pain 12 , 591–599 (2007).
Narita, M. et al. Chronic pain induces anxiety with concomitant changes in opioidergic function in the amygdala. Neuropsychopharmacology 31 , 739–750 (2006).
Suzuki, T. et al. Experimental neuropathy in mice is associated with delayed behavioral changes related to anxiety and depression. Anesth. Analg. 104 , 1570–1577 (2007).
Hasnie, F. S. et al. Further characterization of a rat model of varicella zoster virus-associated pain: relationship between mechanical hypersensitivity and anxiety-related behavior, and the influence of analgesic drugs. Neuroscience 144 , 1495–1508 (2007).
Stevenson, G. W., Bilsky, E. J. & Negus, S. S. Targeting pain-suppressed behaviors in preclinical assays of pain and analgesia: effects of morphine on acetic acid-suppressed feeding in C57BL/56J mice. J. Pain 7 , 408–416 (2006).
Malick, A., Jakubowski, M., Elmquist, J. K., Saper, C. B. & Burstein, R. A neurochemical blueprint for pain-induced loss of appetite. Proc. Natl Acad. Sci. USA 98 , 9930–9935 (2001).
Millecamps, M., Etienne, M., Jourdan, D., Eschalier, A. & Ardid, D. Decrease in non-selective, non-sustained attention induced by a chronic visceral inflammatory state as a new pain evaluation in rats. Pain 109 , 214–224 (2004).
Vierck, C. J., Yezierski, R. P. & Light, A. R. Long-lasting hyperalgesia and sympathetic dysregulation after formalin injection into the rat hind paw. Neuroscience 153 , 501–506 (2008).
Cain, C. K., Francis, J. M., Plone, M. A., Emerich, D. F. & Lindner, M. D. Pain-related disability and effects of chronic morphine in the adjuvant-induced arthritis model of chronic pain. Physiol. Behav. 62 , 199–205 (1997).
Messaoudi, M. et al. Behavioral evaluation of visceral pain in a rat model of colonic inflammation. Neuroreport 10 , 1137–1141 (1999).
Rodriguez, R. & Pardo, E. G. Drug reversal of pain induced functional impairment. Arch. int. Pharmacodyn. Ther. 172 , 148–160 (1968).
Houghton, A. K., Kadura, S. & Westlund, K. N. Dorsal column lesions reverse the reduction of homecage activity in rats with pancreatitis. Neuroreport 8 , 3795–3800 (1997).
Bon, K., Lichtensteiger, C. A., Wilson, S. G. & Mogil, J. S. Characterization of cyclophosphamide cystitis, a model of visceral and referred pain in the mouse: species and strain differences. J. Urol. 170 , 1008–1012 (2003).
Zhao, M.-G. et al. Enhanced presynaptic neurotransmitter release in the anterior cingulate cortex of mice with chronic pain. J. Neurosci. 26 , 8923–8930 (2006).
Monassi, C. R., Bandler, R. & Keay, K. A. A subpopulation of rats show social and sleep-waking changes typical of chronic neuropathic pain following peripheral nerve injury. Eur. J. Neurosci. 17 , 1907–1920 (2003).
Farabollini, F., Giordano, G. & Carli, G. Tonic pain and social behavior in male rabbits. Behav. Brain Res. 31 , 169–175 (1988).
Andersen, M. L. & Tufik, S. Sleep patterns over 21-day period in rats with chronic constriction of sciatic nerve. Brain Res. 984 , 84–92 (2003).
Guevara-Lopez, U., Ayala-Guerrero, F., Covarrubias-Gomez, A., Lopez-Munoz, F. J. & Torres-Gonzalez, R. Effect of acute gouty arthritis on sleep patterns: a preclinical study. Eur. J. Pain 13 , 146–153 (2009).
Landis, C. A., Robinson, C. R. & Levine, J. D. Sleep fragmentation in the arthritic rat. Pain 34 , 93–99 (1988).
Munro, G., Erichsen, H. K. & Mirza, N. R. Pharmacological comparison of anticonvulsant drugs in animal models of persistent pain and anxiety. Neuropharmacology 53 , 609–618 (2007).
Kontinen, V. K., Ahnaou, A., Drinkenburg, W. H. & Meert, T. F. Sleep and EEG patterns in the chronic constriction injury model of neuropathic pain. Physiol. Behav. 78 , 241–246 (2003).
Tokunaga, S. et al. Changes of sleep patterns in rats with chronic constriction injury under aversive conditions. Biol. Pharm. Bull. 30 , 2088–2090 (2007).
Cryan, J. F. & Holmes, A. The ascent of mouse: advances in modelling human depression and anxiety. Nature Rev. Drug Discov. 4 , 775–790 (2005).
Hasnie, F. S., Wallace, V. C. J., Hefner, K., Holmes, A. & Rice, A. S. C. Mechanical and cold hypersensitivity in nerve-injured C57BL/56J mice is not associated with fear-avoidance- and depression-related behaviour. Br. J. Anaesth. 98 , 816–822 (2007).
Kontinen, V. K., Kauppila, T., Paananen, S., Pertovaara, A. & Kalso, E. Behavioural measures of depression and anxiety in rats with spinal nerve ligation-induced neuropathy. Pain 80 , 341–346 (1999).
Jourdan, D., Ardid, D. & Eschalier, A. Automated behavioural analysis in animal pain studies. Pharmacol. Res. 43 , 103–110 (2001).
Roughan, J. V., Wright-Williams, S. L. & Flecknell, P. A. Automated analysis of postoperative behaviour: assessment of HomeCageScan as a novel method to rapidly identify pain and analgesic effects in mice. Lab. Anim. 43 , 17–26 (2009). The future of algesiometry in the laboratory animal?
Chesler, E. J., Wilson, S. G., Lariviere, W. R., Rodriguez-Zas, S. L. & Mogil, J. S. Influences of laboratory environment on behavior. Nature Neurosci. 5 , 1101–1102 (2002).
Ueta, K. et al. Long-term treatment with the Na + -glucose cotransporter inhibitor T-1095 causes sustained improvement in hyperglycemia and prevents diabetic neuropathy in Goto-Kakizaki rats. Life Sci. 76 , 2655–2668 (2005).
Cimino-Brown, D. et al. Physiologic and antinociceptive effects of intrathecal resiniferatoxin in a canine bone cancer model. Anesthesiology 103 , 1052–1059 (2005).
Mizisin, A. P., Shelton, G. D., Burgers, M. L., Powell, H. C. & Cuddon, P. A. Neurological complications associated with spontaneously occurring feline diabetes mellitus. J. Neuropathol. Exp. Neurol. 61 , 872–884 (2002).
Mao, J. NMDA and opioid receptors: their interactions in antinociception, tolerance and neuroplasticity. Brain Res. Rev. 30 , 289–304 (1999).
Elliott, K., Kest, B., Man, A., Kao, B. & Inturrisi, C. E. N-Methyl- D -aspartate (NMDA) receptors, mu and kappa opioid tolerance, and perspectives on new analgesic drug development. Neuropsychopharmacology 13 , 347–356 (1995).
Kozela, E. & Popik, P. The effects of NMDA receptor antagonists on acute morphine antinociception in mice. Amino Acids 23 , 163–168 (2002).
Kemp, J. A. & McKernan, R. M. NMDA receptor pathways as drug targets. Nature Neurosci. 5 , 1039–1042 (2002).
Chizh, B. A., Headley, P. M. & Tzschentke, T. M. NMDA receptor antagonists as analgesics: focus on the NR2B subtype. Trends Pharmacol. Sci. 22 , 636–642 (2001).
Monck, N. Morphine/dextromethorphan - endo: E 3231, Morphidex. Drugs R D 4 , 55–56 (2003).
Lipa, S. M. & Kavaliers, M. Sex differences in the inhibitory effects of the NMDA antagonist, MK-801, on morphine and stress-induced analgesia. Brain Res. Bull. 24 , 627–630 (1990).
Nemmani, K. V. S., Grisel, J. E., Stowe, J. R., Smith-Carliss, R. & Mogil, J. S. Modulation of morphine analgesia by site-specific N -methyl- D -aspartate receptor antagonists: dependence on sex, site of antagonism, morphine dose, and time. Pain 109 , 274–283 (2004).
Grisel, J. E., Allen, S., Nemmani, K. V. S., Fee, J. R. & Carliss, R. The influence of dextromethorphan on morphine analgesia in Swiss Webster mice is sex-specific. Pharmacol. Biochem. Behav. 81 , 131–138 (2005).
Craft, R. M. & Lee, D. A. NMDA antagonist modulation of morphine antinociception in female vs. male rats. Pharmacol. Biochem. Behav. 80 , 639–649 (2005).
Bryant, C. D., Eitan, S., Sinchak, K., Fanselow, M. S. & Evans, C. J. NMDA receptor antagonism disrupts the development of morphine analgesic tolerance in male, but not female C57BL/56J mice. Am. J. Physiol. Regul. Integr. Comp. Physiol. 291 , R315–R326 (2006).
Holtman, J. R. Jr., Jing, X. & Wala, E. P. Sex-related differences in the enhancement of morphine antinociception by NMDA receptor antagonists in rats. Pharmacol. Biochem. Behav. 76 , 285–293 (2003).
Holtman, J. R. Jr. & Wala, E. P. Characterization of morphine-induced hyperalgesia in male and female rats. Pain 114 , 62–70 (2005).
Juni, A., Klein, G., Kowalczyk, B., Ragnauth, A. & Kest, B. Sex differences in hyperalgesia during morphine infusion: effect of gonadectomy and estrogen treatment. Neuropharmacology 54 , 1264–1270 (2008).
Levine, J. D., Fields, H. L. & Basbaum, A. I. Peptides and the primary afferent nociceptor. J. Neurosci. 13 , 2273–2286 (1993).
Nakamura-Craig, M. & Gill, B. K. Effect of neurokinin A, substance P and calcitonin gene related peptide in peripheral hyperalgesia in the rat paw. Neurosci. Lett. 124 , 49–51 (1991).
Gamse, R. & Saria, A. Nociceptive behavior after intrathecal injections of substance P, neurokinin A and calcitonin gene-related peptide. Neurosci. Lett. 70 , 143–147 (1986).
Pedersen-Bjergaard, U. et al. Calcitonin gene-related peptide, neurokinin A and substance P: effects on nociception and neurogenic inflammation in human skin and temporal muscle. Peptides 12 , 333–337 (1991).
Saxen, M. A., Welch, S. P. & Dewey, W. L. The mouse paw withdrawal assay: a method for determining the effect of calcitonin gene-related peptide on cutaneous heat nociceptive latency time. Life Sci. 53 , 397–405 (1993).
Mogil, J. S. et al. Variable sensitivity to noxious heat is mediated by differential expression of the CGRP gene. Proc. Natl Acad. Sci. USA 102 , 12938–12943 (2005).
MacIntyre, L. C. et al. Sex-dependent fear of human experimenters and facsimiles thereof in laboratory mice. Soc. Neurosci. Abstr. 33 (2007).
Download references
Acknowledgements
The author would like to thank G. Bennett and the anonymous reviewers for helpful comments on this manuscript.
Author information
Authors and affiliations.
Department of Psychology and Alan Edwards Centre for Research on Pain, McGill University, Montreal, H3A 1B1, Quebec, Canada
- Jeffrey S. Mogil
You can also search for this author in PubMed Google Scholar
Related links
Further information.
Jeffrey S. Mogil's homepage
Pain Genes Database
The ratio of the minimum dose of a drug that causes toxic effects to the therapeutic dose, used as a relative measure of drug safety.
Pain arising as a direct consequence of a lesion or disease affecting the somatosensory system.
Measures of involuntary movements made in response to a stimulus. For example, the nociceptive withdrawal reflex is a spinal (segmental) reflex intended to protect the body from potentially damaging noxious stimuli. Spino-bulbospinal reflexes, lost after spinal transection but preserved after decerebration, include licking, guarding, vocalizing and jumping.
Measures of behaviours that require spinal-cerebrospinal integration, which are lost after decerebration. The use of operant measures specifically requires a learned, motivated behaviour that terminates exposure to the noxious stimulus.
A specific physical or chemical entity used to measure or indicate the effects or progress of a disease or condition.
A property of a model that seems to obviously ('on its face') measure what it is supposed to measure.
A sequence-specific gene-silencing tool used for RNA interference. Small interfering RNAs are short fragments of synthetic double-stranded RNA with 21–23 pairs of nucleotides that have sequence specificity to the gene of interest. They trigger degradation of the target RNA, thereby creating a partial loss-of-function by decreasing the amount of translatable RNA.
An increased response to a stimulus which is normally painful.
Somatic or visceral pain processed by a normal, unaltered nervous system.
A statistical technique used to identify particular regions of the genome containing DNA variants responsible for between-strain variation on a quantitative (complex) trait.
An assay of acute and tonic pain in which a dilute solution of formalin (37% w/w formaldehyde) is injected into the dorsal or plantar hindpaw. Formalin produces two 'phases' of pain behaviour separated by a quiescent period: the early phase is probably due to direct activation of nociceptors through TRPA1 channels; the second phase is due to ongoing inflammatory input and central sensitization.
Pain resulting from a stimulus that does not normally provoke pain.
Nylon monofilaments that, when pressed against tissue until they bend, exert a calibrated amount of force. They are used to measure mechanical sensibility.
A behavioural task during which a subject learns to associate an experience with a specific physical environment. A subject will choose to spend more time in an environment in which it previously had a rewarding experience (for example, an analgesic drug) and less time in an environment in which it had an aversive experience (for example, inflammatory pain).
A catalogue of discrete behaviours displayed by an organism.
The attribution of human characteristics to non-human organisms. Whereas this attribution is often mistaken, people can also make the opposite error, anthropodenial, denying our commonalities with other species.
Rights and permissions
Reprints and permissions
About this article
Cite this article.
Mogil, J. Animal models of pain: progress and challenges. Nat Rev Neurosci 10 , 283–294 (2009). https://doi.org/10.1038/nrn2606
Download citation
Published : 04 March 2009
Issue Date : April 2009
DOI : https://doi.org/10.1038/nrn2606
Share this article
Anyone you share the following link with will be able to read this content:
Sorry, a shareable link is not currently available for this article.
Provided by the Springer Nature SharedIt content-sharing initiative
This article is cited by
Lncrna anxa10-203 enhances mc1r mrna stability to promote neuropathic pain by recruiting dhx30 in the trigeminal ganglion.
- YanYan Zhang
The Journal of Headache and Pain (2024)
Audible pain squeaks can mediate emotional contagion across pre-exposed rats with a potential effect of auto-conditioning
- Julian Packheiser
- Christian Keysers
Communications Biology (2023)
Red hair and pain sensitivity: insights into genomics of pain?
- Hannah R. Williams
- Jaideep J. Pandit
Anesthesiology and Perioperative Science (2023)
- Katelyn E. Sadler
- Cheryl L. Stucky
Nature Reviews Neuroscience (2022)
Translational science: a survey of US biomedical researchers’ perspectives and practices
- Rebecca L. Walker
- Katherine W. Saylor
- Jill A. Fisher
Lab Animal (2022)
Quick links
- Explore articles by subject
- Guide to authors
- Editorial policies
Sign up for the Nature Briefing newsletter — what matters in science, free to your inbox daily.


- About Journal overview Indexed Copyright policy Contact us
- Information for Authors Areas and categories Submitting a manuscript Selection of manuscripts Preparing a revised paper and relative materials Style and format Publication charges Contact information
- Editorial Board Present Editorial Board Youth Editorial Board
- Subscription
- Notice Notice
Center for Translational Medicine Research on Sensory-Motor Diseases, Yan’an University, Yan’an 716000, China
This work was supported by grants from The National Natural Science Foundation of China (82074564,81772451,81860410).
Visceral pain is pathological pain and common clinical symptoms sourced from internal organs exposed to noxious stimuli such as mechanical stretch, inflammation, spasm, and ischemia. Compared with somatic pain, the mechanisms of induction and maintenance of viseral pain and its modulation are more complex. It has been demonstrated that peripheral mechanisms, i.e . peripheral inflammation, variations of the number and function of ion channels/receptors, central sensitization, and imbalanced descending controls: inhibition and facilitation are all involved in visceral pain. Therefore, clarifying mechanisms of visceral pain is important in basic pain research as well as in the clinic. For the in-depth study of the complex mechanisms underlying visceral pain, the choice and establishment of experimental animal models that mimic the pathophysiological characteristics of clinical visceral diseases is an essential prerequisite for the exploration of visceral pain. One difficulty in establishing animal model of visceral pain is that the experimental animal model cannot completely simulate the pathophysiological characteristics of clinical visceral diseases, and visceral diseases may be accompanied by a series of complications. In addition, repeatability, easiness and economy should be considered during the establishment of the animal model of visceral pain. At present, there are various animal models and classification methods of visceral pain, during which no authoritative classification of visceral pain animal models is validated. Currently, animal model of visceral pain is classified according to the method of visceral stimulation, such as inflammatory factors, mechanical dilative, ischemic, and electrical stimulation. Besides, visceral pain model can be classified according to the location of occurrence, i.e . heart, gastroduodenum, pancreas, small intestine, colorectal, ureter, bladder and uterus. The establishment of an ideal experimental animal model in line with the pathophysiological characteristics of clinical visceral diseases is of great significant and critical to explore the mechanisms of induction, maintenance, and regulation in visceral pain, and to further screen the therapeutic drugs for related visceral diseases. The aim of current review is to summary the preparation and characteristics of common experimental animal visceral pain models, and provide relative documents for basic researchers and clinicians to select appropriate animal models in exploring visceral pain, better understanding the associated mechanisms, and providing available therapeutic strategy.
Get Citation
WANG Wen-Wen, LEI Jing, YOU Hao-Jun.Review: Research Progress of Experimental Animal Models of Visceral Pain[J]. Progress in Biochemistry and Biophysics,2022,49(5):858-866
Article Metrics
- Received: January 09,2022
- Revised: April 15,2022
- Accepted: April 15,2022
- Online: May 20,2022
- Published: May 20,2022
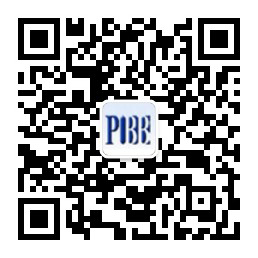
® 2024 All Rights Reserved ICP:京ICP备05023138号-1 京公网安备 11010502031771号
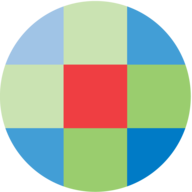
- Subscribe to journal Subscribe
- Get new issue alerts Get alerts
Secondary Logo
Journal logo.
Colleague's E-mail is Invalid
Your message has been successfully sent to your colleague.
Save my selection
Experimental Animal Models of Muscle Pain and Analgesia
Kehl, Lois J. 1 ; Fairbanks, Carolyn A. 2 3 4
Departments of 1 Anesthesiology and Pain Medicine, 2 Pharmaceutics, 3 Pharmacology, and 4 Neuroscience, University of Minnesota, Minneapolis
Accepted for publication: March 24, 2003.
Address for correspondence: Carolyn A. Fairbanks, Ph.D., Department of Pharmaceutics, University of Minnesota, 9–177 Weaver Densford Hall, 308 Harvard Street S.E., Minneapolis, MN 55455 (E-mail: [email protected] ).
KEHL, L. J., and C. A. FAIRBANKS. Experimental animal models of muscle pain and analgesia. Exerc. Sport Sci. Rev. , Vol. 31, No. 4, pp. 188–194, 2003. Muscle pain is a prevalent clinical problem but can be difficult to treat because relatively little is known about nervous system mechanisms that mediate and modulate it. This review profiles four new animal models of muscle and deep tissue pain currently being used to elucidate mechanisms of muscle pain and analgesia.
Alleviation of muscle pain is challenging because of the minimal information on biological cause. In vivo models may elucidate such mechanisms.
INTRODUCTION
Clinically treated pain states principally involve pain of muscle, joint, or visceral origin. Unfortunately, most of our knowledge regarding pain mechanisms is based on studies of cutaneous tissue. Relatively little is known about the activation of joint or visceral nociceptors, and perhaps least of all about muscle nociceptors. This is largely because of the multiple inputs that influence muscle sensation, including muscle spindles, tendon receptors, joint receptors, and skin receptors as well as CNS processing of these inputs. The complexity of these many inputs complicates the process of elucidating the mechanisms involved in transmitting and modulating pain from the deep tissues of the musculoskeletal system. In practical terms, this lack of knowledge reflects a real void in the understanding needed to treat musculoskeletal pain effectively, even though it is one of the most frequent symptoms for which patients seek medical treatment.
In the clinical setting, pain may be refractory to pharmaceutical interventions or patients may refuse to take pain medication because of adverse side effects. Furthermore, pain originating from different tissues or as the result of different disease processes responds differently to various drugs or therapies ( e.g. , ultrasound, transcutaneous electrical nerve stimulation, iontophoresis) used for pain management for reasons that may not be fully understood. The mechanistic differences governing the multiplicity of clinical pain conditions has received recognition relatively recently by scientists studying nociception and analgesia. Refinement of animal models and methods for assessing pain thresholds under varied conditions has greatly expanded the potential for identifying those differences.
Previously, standard animal models used to study pain mechanisms relied on various nerve injuries and tissue inflammation of the paw to evaluate pain responses and the efficacy of analgesics. Most standard dependent measures assess the subject’s response to stimulation of nerve endings that innervate the epidermis (of the paw, for example). However, pain is a response to stimulation of nerve endings in a variety of tissues, not merely epidermal tissue. The impact of exercise and movement on pain thresholds and efficacy of analgesics has received limited attention in basic science investigations. Development of several new models that specifically assess hypersensitivity caused by noxious stimuli in muscle may help to understand pain originating from muscle. This paper profiles these new animal models that are improving our understanding of muscle hyperalgesia and analgesia. Hyperalgesia is a term the describes the increase in sensitivity to normally noxious stimuli that an organism may experience after inflammation, injury, or another physiologic change that lowers the threshold to pain. Analgesia refers to the reduction in sensitivity to noxious stimuli that an organism detects, often as a result of the administration of pain-relieving drugs, such as morphine.
EXERCISE-INDUCED MUSCLE HYPERALGESIA
Prolonged eccentric exercise is well known to produce muscle injury, inflammation, and delayed-onset muscle soreness (DOMS) in humans ( 3 ). DOMS is characterized by muscle weakness and infiltration of inflammatory cells into the affected tissues. Consequently, to elucidate mechanisms of human muscle pain, some investigators have attempted to reproduce this phenomenon in animal models.
One such animal model used eccentric treadmill exercise to model DOMS in rats as follows. Sprague Dawley rats performed one of two exercise protocols: (a) in the experimental group, rats ran on a treadmill (60 m·min −1 at an approximately 30° downhill incline for 15 min, twice in 2 h; or (b) in the control group, rats were placed on a treadmill at a walking pace (6 m·min −1 for 15 min, twice in 2 h). After this exercise protocol, animals were tested at intervals over the next several hours for their capacity to perform resisted flexion of their forelimbs against a force transducer (also known as forelimb grip force ) ( 4 ). In the experimental group, prolonged eccentric exercise resulted in a significant time-dependent reduction in forelimb grip force over the 48-h observation period ( P < 0.005) with peak reduction in forearm grip force occurring 18 to 24 h after exercise ( Fig. 1 ). Reduction in grip force was reversed by the μ opioid agonist levorphanol (3 mg·kg −1 intraperitoneally [i.p.]; P < 0.05) but not levorphanol coadministered with the opioid antagonist naltrexone (3 mg·kg −1 i.p.; Fig. 2 ). Because opioids are not known directly to affect ( e.g. , enhance) muscle force, the observed reversal of grip force reduction by the opioid is consistent with an analgesic effect rather than the result of increased muscle force production. This result provided evidence that the eccentric exercise protocol described above produced muscle hyperalgesia. Unfortunately, because of the labor-intensive nature of this protocol and because the magnitude of hyperalgesia (measured by reduction in grip force) was not large enough to discriminate drug effects in pharmacologic experiments, this model for DOMS was not developed further.

CARRAGEENAN-INDUCED MUSCLE HYPERALGESIA
A second experimental paradigm reproduces the muscle inflammation associated with DOMS without the possible confound that eccentric exercise-induced muscle fatigue or analgesia could produce. This model ( 5 ) uses bilateral intramuscular injections of carrageenan (4 mg·75 μL −1 phosphate buffered saline [PBS]) into the triceps muscles of rodents to produce a localized inflammatory response similar to the inflammation produced during eccentric exercise. Carrageenan is an extract of seaweed that causes localized inflammation when administered to various tissues and is used in some standard animal models of inflammatory pain as a pain-producing stimulus. In this model of muscle hyperalgesia, the amount of forelimb force ( i.e. , forelimb grip force) exerted by rodents after carrageenan injection is compared with force exerted before injection and used as a behavioral dependent measure of hyperalgesia.
Several studies have shown that patients with chronic muscle pain exert reduced force with their tender muscles. Furthermore, reduced muscle strength strongly correlates with increased numbers of tender points in pain patients. This reduction in force exertion by painful muscles most likely is the result of a reduction in the activity of agonist muscles and an increase in the activity of antagonist muscles. These reports and others formed the basis for choosing reduced forelimb grip force as a behavioral index of hyperalgesia in the rodent model of muscle hyperalgesia described next.
In this model, forelimb grip force measurements are acquired before carrageenan is injected and then at various intervals after carrageenan. Typically, rats exhibit a 160-g reduction in forelimb grip force 12 to 24 h after carrageenan administration, whereas mice exhibit a 40-g peak reduction 48 h after carrageenan administration. Several experimental results validated the reduction of forelimb grip force after intramuscular carrageenan administration as a measure of muscle hyperalgesia ( 5,15 ). In particular, the peak reduction in forelimb grip force was reversed within 30 min after administration of several drug classes ( i.e. , the opioids levorphanol [1 mg·kg −1 i.p. in rats] and morphine [ED 50 5.3 mg·kg −1 i.p. in mice], the nonsteroidal antiinflammatory drug indomethacin [4 mg·kg −1 i.p. in rats], and the steroid dexamethasone [4 mg·kg −1 i.p. in rats]) used clinically to treat muscle pain. If the reduction in grip force had been the result of muscle fatigue, analgesic drugs would not have been able to reverse this effect. Furthermore, if muscle injury had been responsible for the reduced forelimb force, then this effect would not have been reversible within 30 min after drug administration. Collectively, these studies provide support that reduction in forelimb grip force is a valid assessment of muscle hyperalgesia.
Behavioral models of nociception are widely used efficiently to screen potential analgesic compounds for therapeutic efficacy in the treatment of human pain. Although not always mirroring naturally occurring painful conditions ( e.g. , tail flick test, formalin test, intrathecal substance P test), the value of these models lies in their capacity to predict reliably which pharmacologic compounds will be analgesic when administered to pain patients. Therefore, because the model of carrageenan-evoked muscle hyperalgesia reliably evokes a behavioral response reported by muscle pain patients ( i.e. , reduction in grip force), is reversed by several clinically useful analgesic agents, demonstrates an amplitude of effect that allows pharmacologic testing, and shares an inflammatory component with DOMS, this model has gained popularity.
TUMOR-INDUCED MOVEMENT-RELATED PAIN
Another type of movement-related pain, termed breakthrough pain , is a common problem for patients with cancer pain and is considered to be a predictor of poor response to conventional analgesic agents. Recently, a new animal model of movement-evoked cancer pain was developed to facilitate the investigation of mechanisms contributing to breakthrough pain ( 15 ). It uses the implantation of NCTC 2472 sarcoma cells into the humerus bones of C3H/He mice to simulate the development of osteolytic sarcoma, a form of bone cancer, in these animals. As in the carrageenan model described above, the investigators inferred the degree of insult-induced, movement-related hyperalgesia from changes in the level of peak force exerted by these animals’ forelimbs using the grip force assay.
In addition, to determine whether secondary mechanical allodynia of the forepaws contributed to the reductions in grip force observed in this assay, cutaneous forepaw hypersensitivity was evaluated using von Frey filaments. von Frey filaments are a series of approximately 2-cm long nylon monofilaments, similar to fishing line, with increasing diameters that bend when a known amount of force (1–358 mN) is applied to them. During a typical experiment, mice are placed on a wire mesh platform and are allowed to acclimate to their surroundings for a minimum of 15 min before testing. von Frey filaments delivering varying levels of force (1–15 mN is the typical range for mice) corresponding to varying diameters of the filament are applied to the point of bending to the plantar surface of the paw. Each test stimulation lasts approximately 3 s. When the stimulus is of sufficient force, the rodent licks, withdraws, or shakes the paw, or a combination thereof; this action represents the behavioral endpoint. The stimulus terminates completely after 1 to 3 s or immediately after paw withdrawal. Based on the forces at which the animal withdraws, a threshold can be calculated to predict the force at which an animal would withdraw 50% of the time, which is somewhat analogous to an ED 50 dose in pharmacology. Normal rats do not respond even to high forces delivered by von Frey monofilaments applied to the point of bending (normal mice respond at 10 mN). Rats subjected to inflammation or nerve injury will withdraw to low forces (approximately 115 mN, injured mice at 1 mN). This is considered a manifestation of mechanical hypersensitivity or hyperalgesia indicating that the thresholds to activation of the pain pathways have been lowered. The reduction in the force that elicits this withdrawal is used as a measure of hyperalgesia.
Using this model of movement-evoked pain, Wacnik et al. ( 15 ) performed a series of experiments to compare the relative effectiveness of two different analgesic agents for reversing tumor-evoked and carrageenan-evoked deep tissue hyperalgesia. The first study evaluated the analgesic potency of the μ opioid agonist morphine. Behavioral indices of hyperalgesia ( i.e. , plantar forepaw von Frey fiber testing and peak forelimb grip force) were measured at baseline, before injection of tumor cells (bilateral humeral injections of 2 × 10 5 2472 cells in a volume of 10 μL PBS) or carrageenan (bilateral injections of 4–8% carrageenan in 40 μL PBS to the triceps), and repeated at various intervals up to 10 d (tumor) or 17 d (carrageenan) after insult. Morphine was administered intraperitoneally at the time when peak hyperalgesia was present in both animal models (10 d after tumor cell implantation and 48 h after carrageenan injection), and grip force was measured 30 min later. In this study, 3 to 30 mg·kg −1 morphine dose dependently returned grip force measurements toward baseline levels in both tumor-bearing (ED 50 19.8 mg·kg −1 ) and carrageenan-injected (ED 50 5.3 mg·kg −1 ) mice. Both tumor cell implantation and injection of 4% carrageenan produced apparent forelimb hyperalgesia without mechanical allodynia in the forepaws, whereas 6% and 8% carrageenan did evoke significant cutaneous allodynia of the forepaws. Control groups receiving identical implants, injections of PBS, or no treatment at all did not manifest this forelimb hyperalgesia.
In a follow-up study, Kehl et al. ( 6 ) used a very similar experimental paradigm to compare the analgesic potency of the cannabinoid agonist WIN55,212–2 for reversing tumor- and carrageenan-evoked hyperalgesia. This compound acts nonselectively at two subtypes of cannabinoid receptors: CB1 receptors, located primarily in the CNS and on primary afferent fibers, and CB2 receptors, located primarily on immune cells. In a series of experiments, these investigators evaluated whether WIN55,212–2 dose dependently reversed tumor- and carrageenan-evoked movement-related hyperalgesia and which receptor subtypes participated in the cannabinoid’s analgesic effects. The results of this study indicated that WIN55,212–2 is approximately four times more potent in reversing carrageenan-evoked hyperalgesia (ED 50 = 5.6 mg·kg −1 ) than tumor-evoked hyperalgesia (ED 50 = 23.3 mg·kg −1 ). Furthermore, in the cancer pain model, WIN 55,212–2’s antihyperalgesic effect was partially blocked by pretreatment with the CB1 (SR141716A) but not the CB2 (SR144528) receptor antagonist. In contrast, both the CB1 and CB2 receptor antagonists blocked antihyperalgesic effects of WIN55,212–2 in the carrageenan model of muscle hyperalgesia. These results provide evidence that CB1 receptors participate in the antihyperalgesia produced by WIN55,212–2 in the cancer pain model but that both receptor subtypes contribute to the antihyperalgesia observed in the carrageenan model that uses an inflammatory pain stimulus. These data provide evidence that cannabinoids differentially modulate tumor- and carrageenan-evoked hyperalgesia and suggest that differences in underlying mechanisms may exist between these two models of deep tissue pain.
Collectively, the studies described above provide evidence that the humeral implantation of osteolytic sarcoma cells in conjunction with forelimb grip force testing provides an animal representation of the movement-related hyperalgesia, or breakthrough pain, frequently associated with cancer. This animal model likely will provide a useful tool to assist in discerning the peripheral and central mechanisms underlying pain that accompanies bone metastases and will allow testing of new analgesic agents for management of cancer pain.
ACID-INDUCED MUSCLE SORENESS
A third approach for modeling muscle hypersensitivity in rodents takes advantage of the observations that tissue pH decreases after inflammation and isometric exercise. This approach is supported further by observations in human clinical experiments that low pH (5.2) evokes some mild detectable muscle pain sensations. Sluka et al. ( 12 ) characterized the nociceptive responses of rodents subjected to injections of a wide range of low pH saline in the gastrocnemius muscle. Halothane-anesthetized male Sprague-Dawley rats (250–400 g) were administered 100 μL of sterile saline acidified to a pH of either 4, 5, 6, or 7.2 (nonacidic). These injections reduce the pH of the injected tissue to a level within the pH range that occurs after tissue injury, inflammation, and exercise. These injections were repeated at different intervals ranging from 2 to 10 d. When spaced between 2 and 5 d apart, injections of pH 4.0 saline will cause a long-lasting (30 d) mechanical (but not thermal) hyperalgesia. Mechanical hypersensitivity was assessed using the von Frey monofilament method. The muscle hypersensitivity in rodents does not appear to be accompanied by inflammation or necrosis (determined by histologic examination). Consequently, Sluka et al. currently are investigating the mechanistic source of the observed acid-induced muscle hypersensitivity. Injection of low pH saline into the gastrocnemius muscle could act directly on a newly discovered ion channel thought to be involved in pain signaling ( 10 ). Acid-sensing ion channels have been localized to small and medium primary afferent neurons and in synaptic terminals in the superficial dorsal horn, a region in the spinal cord that mediates pain signaling. Activation of these channels results in electrical currents that are consistent with those that are thought to signal pain. Decreasing pH in the region of primary afferent peripheral terminals in the gastrocnemius muscle could activate this channel and could drive an electrical signal of pain. Evidence to support this hypothesis was shown in a study where genetically altered mice that do not have the gene for the ASIC channel found in the dorsal root ganglion (DRASIC) were tested to see if they would develop mechanical hyperalgesia in response to injection of pH 4.0 saline. Normal (DRASIC-containing) litter mate controls to the knock-out mice did, as expected, experience mechanical hypersensitivity after injection of acidic (pH 4.0) saline. In contrast, the DRASIC-KO mice experienced some mechanical hypersensitivity compared with preinjection responses; however, the magnitude of the hypersensitivity was significantly less than their normal litter mate counterparts. This result suggests that DRASIC may, in fact, contribute to acid-induced changes in muscle soreness, at least in this murine model ( 10 ). The mechanical hyperalgesia manifested by the acidified saline injections is alleviated by administration of gold standard analgesics such as the μ opioid receptor-selective agonists morphine and DAMGO (D-Ala(2), Me-Phe(4),Gly(ol)(5)]enkephalin) as well as the delta opioid receptor-selective agonist SNC-80 ( 13 ). This evidence supported the assertion that the model reflects a true nociceptive event. Furthermore, antagonism of N-methyl-D-aspartate (NMDA) receptors and non-NMDA glutamate receptors by administration of APV (D-(-)-2-amino-5-phosphonovalerate) and NBQX (2,3-dioxo-6-nitro-1,2,3,4-tetrahydrobenzo-[f]-quinoxaline-7-sulfonamide), respectively, 1 week after the second intramuscular injection of acidified saline (pH 4.0) reversed mechanical hypersensitivity ( 11 ). These results indicate that, similar to other chronic pain syndromes, NMDA and non-NMDA receptors contribute to the maintenance of mechanical hyperalgesia in this model. This report also suggests that the intramuscular injection of acidified saline model invokes plastic changes within the dorsal horn of the spinal cord that may account for the duration of this chronic mechanical hypersensitivity.
EXERCISE-INDUCED ANALGESIA
Exercise has been reported anecdotally to increase pain thresholds in patients. Biochemical support for this phenomenon has been provided by animal models of swimming ( 14 ). Natural (endogenous) pain relievers (analgesics) that are synthesized and localized in the brain may account for the increase in pain threshold in subjects that are exercised. There are several classes of analgesic neurotransmitters that likely contribute to this phenomenon. The first class includes the “morphine-like” or opioid peptides. These peptides are called endorphin, endomorphin, enkephalin, and dynorphin. They act on proteins that reside in the lipid bilayer of the cell membranes of neurons in the brain and in the spinal cord. These proteins are known as the μ, δ, and κ opioid receptors. Activation of these receptors (by morphine or by these endogenous neurotransmitters) causes a neuron to hyperpolarize such that electrical signals of pain cannot be transmitted through the neuronal circuitry to the brain. Epinephrine and norepinephrine (catecholamines) are neurotransmitters that act on a separate class of neuronal proteins to inhibit pain signaling. When given in combination, norepinephrine and the opioid peptides will “synergize” to give a superadditive analgesic effect that is a level of analgesia greater than the sum of analgesia achieved by the two drugs given individually.
In animal models of exercise, it has been shown that concentrations of β-endorphin ( 14 ) and catecholamines ( 9 ) are increased centrally and peripherally in exercised rodents compared with nonexercised controls. Similarly, plasma β-endorphin ( 2 ) and norepinephrine ( 8 ) levels have been observed to rise significantly in humans after acute or chronic exercise, respectively. It is, therefore, possible that the reported exercise-induced analgesia or the increased pain thresholds are attributable to increased basal β-endorphin levels acting on μ or δ opioid receptors to exert an analgesic effect alone or to potentiate exogenously administered analgesics. Recently two more endogenous opioids have been identified: the discovery of mu opioid receptor selective tetrapeptides endomorphins 1 and 2 raise the possibility that those entities also participate in these processes (although this hypothesis remains to be tested). It is likely that further investigations will be conducted to assess the impact of these molecules.
EXERCISE-INDUCED REDUCTION IN ANALGESIA
In contrast to the reports of exercise-induced analgesia, there may be some instances where chronic participation in exercise may, in fact, decrease the efficacy of analgesics. In several rodent models of exercise including wheel running ( 7 ) and swimming ( 1 ), the analgesic potency of exogenously applied morphine was decreased. Several possibilities may explain this phenomenon. It is conceivable that chronic upregulation of opioid peptides ( e.g. , enkephalin, endorphin, and endomorphin) or norepinephrine could cause tolerance at their respective cell membrane receptors. Tolerance could result from a decrease in available receptor number or a decrease in the signal transduction machinery such as is depicted in Figure 3 . In these studies, it was observed that acutely induced exercise did not reveal tolerance to opioid-induced analgesia and that chronic exposure to exercise was required to observe this effect. However, we have conducted a study that may have detected an acutely induced analgesic tolerance after prolonged exercise during a single session on the rotorod (Ugo Basile, Italy).

MILD ACUTE ANALGESIC TOLERANCE AFTER EXERCISE IN MICE
Substance P (SP) is a neurotransmitter that is released in the spinal cord in response to noxious stimuli at the periphery. SP carries the pain signal to secondary neurons that reside in the spinal cord and subsequently carry the information about pain to higher brain centers. Direct intrathecal injection (5 μL) of exogenous SP (15 ng) in Institute of Cancer Research (ICR) mice results in biting, licking, and scratching behaviors directed to the hind limb for 1 min after the injection. These behaviors can be counted and used for quantification. SP-induced behaviors are dose dependently alleviated by opioid and α 2 AR agonists in a naloxone-sensitive and idazoxan-sensitive manner, respectively, indicating that this behavior can be used as a model of pain. This model has been approved by the University of Minnesota Institutional Animal Care and Use Committee. The correspondence between results using SP and standard antinociceptive tests ( e.g. , tail flick) support the usefulness of the SP assay for antinociceptive inference. We used the SP assay to assess the analgesic efficacy of low intrathecal doses of two combined α 2 AR adrenergic analgesics, clonidine and dexmedetomidine. These drugs are commonly used in the clinic for the treatment of pain and for anesthesia. To simulate exercise, mice were first trained to walk for 300 s on an accelerating rotorod device. The suspended rotorod (diameter is 3 cm) rotates in space and accelerates from 4 to 40 rotations·min −1 within the 5-min session. The rotorod often is used to assess sedation and motor performance after drug administration. In the first training sessions, the mice fall off (6 inches to the platform) the rotorod within the first minute. After being reintroduced to the rotorod several times, the mice learn to stay on the rotorod for the 5-min training duration. Different strains of mice show different training requirements. ICR mice learn to remain on the rotorod for the 5-min period within two training sessions. C3H mice require three to four training sessions, and a mixed hybrid of C57BL/6 and 129sv mice require five to six training sessions to learn to walk on the rotorod for 5 min. For these experiments, we used ICR mice. After they acquired the skill, they were expected to walk on the accelerating rotorod for 300 s. The rotorod was stopped, and the second session began (initially of slower speed 4 rotations·min −1 ramping up to 40 rotations·min −1 within the 5 min). Therefore, the exertion level was variable during the 30 min that comprised the five sessions of accelerated walking.
Immediately after the end of the 30 min, the mice were given the SP nociceptive behavioral test either without (to establish a baseline control group) or with the analgesic combination of clonidine plus dexmedetomidine (the dose of both drugs was either 0.3 pmole·5 μL −1 or 1 pmole·5 μL −1 ). In animals that had been trained to walk on the rotorod a day earlier but had not been acutely exercised, those two doses of clonidine plus dexmedetomidine demonstrated partial efficacy in inhibiting the SP-evoked behavior ( Fig. 4 ). However, the analgesic efficacy of those two dose combinations was completely abolished in mice that had been acutely exercised (as described above) on the rotorod ( Fig. 4 ). Higher doses of either drug given alone were effective in the acutely exercised mice on the rotorod. We only observed this ablated analgesic efficacy at the very low dose-combination of lower analgesic efficacy doses. Given the fact that in the previous studies the analgesic tolerance was only observed in chronically exercised animals, it is probable that a mild acute tolerance or acute cross-tolerance from potential exercise-induced release of endogenous analgesic neurotransmitters ( e.g. , enkephalin, endomorphins 1–2, endorphin) may be detectable only at very low doses of exogenous analgesics. We have reported previously that acute tolerance to exogenous spinal administration of the endogenous neurotransmitter endomorphins 1–2 can occur in ICR mice, and so it is conceivable that this could be an explanation, but more studies would be needed to test that hypothesis.

CONCLUSIONS
The recognition that “pain” originates from diverse dysfunctional physiologic states is a relatively recent development in the field of pain research. Previously, most information available regarding the transmission and modulation of pain was based on studies performed on cutaneous tissue. However, this new appreciation by basic scientists, that clinical pain states result from a variety of causes, has inspired development of new models specifically to examine pain originating from peripheral ( i.e. , neuropathic pain) or central nervous system ( i.e. , spinal cord injury pain) disruption as well as from viscera ( i.e. , cardiac ischemia, painful bowel distention) and deep tissues ( i.e. , cancer pain, muscle pain, fibromyalgia, arthritis). These and other painful conditions currently are being evaluated for differences that may facilitate specific and targeted treatment strategies.
One excellent example of the value that animal models have for improving clinical pain management is the rapid advances made in neuropathic pain management since the development of a few animal models for neuropathic pain in the late 1980s and early 1990s. These animal models established precedent for Food and Drug Administration approval of new uses for existing drugs ( i.e. , antidepressants) as well as development of new drugs specifically targeted to block pathophysiologic mechanisms specific to neuropathic pain. Unfortunately, our understanding of deep tissue pain mechanisms lags behind. Currently, the best therapeutic result that many patients with chronic deep tissue pain can achieve is only partial relief of symptoms. The inability to manage these chronic pain states fully results in substantial time lost from work for those affected as well as disruption of family function and frequently in depression. In the absence of animal models, determination of the mechanisms underlying muscle pain and exercise-induced analgesia (or diminished analgesia) would not be possible. Development of novel pharmaceutical agents and therapeutic methods that specifically target these painful conditions will increase in precision as these new models are more widely applied in research and as new models expand the experimental repertoire.
Acknowledgments
The authors thank Dr. Ken Hargreaves and Mr. Tom Trempe for their contributions to development of the forelimb grip force assay and the exercise- and carrageenan-evoked muscle hyperalgesia studies described here. A Mentored Scientist Career Development Award (K01-DA00509) provided by the National Institute on Drug Abuse (NIDA) supported CAF’s contribution to this manuscript. A NIDA Scientist Development Award (K21-DA00240) supported development of the models reported by LJK.
- Cited Here |
- PubMed | CrossRef
- View Full Text | PubMed | CrossRef
myalgia; analgesia; pain; exercise
- + Favorites
- View in Gallery


- Our Physicians
- Current Residents
- Program Structure
- Resident Life
- Fellowship Match Results
- Affiliated Hospitals
- Salary And Benefits
- Residency Application Information
- Visiting Medical Student Information
- Current Fellows
- The Postgraduate Experience
- Life on Long Island
- Salary & Benefits
- Fellowship Application Information
- Patient Information
- Donate: Fellowship Program
- Donate: Residency Program
- Donate: Department of Orthopaedics
- Department of Orthopaedics
Acute Postoperative Pain and Dorsal Root Ganglia Transcriptomic Signatures Following Total Knee Arthroplasty (TKA) in Rats: An Experimental Study
Justice Achonu, MD 1 ; David E. Komatsu, PhD 1 ; Sardar MZ Uddin, PhD 1 ; Chris Gordon, BS 2 ; Martha P. Kanjiya, BS 2 ; Diane Bogdan, PhD 2 ; Adriana DiBua 2 , Hira Iftikhar 2 , Amanda Ackermann 2 , Rohan J. Shah 2 , Jason Shieh 3 , Agnieszka B. Bialkowska 3 , and Martin Kaczocha, PhD 2
1 Department of Orthopaedics and Rehabilitation, Stony Brook University
2 Department of Anesthesiology, Stony Brook University
3 Department of Medicine, Stony Brook University
Disclosure Statement: None of the authors has any funding sources, commercial, or financial conflicts of interest to declare.
Total knee arthroplasty (TKA) is the final treatment option for patients with advanced knee osteoarthritis (OA) . Unfortunately, TKA surgery is accompanied by acute postoperative pain that is more severe than arthroplasty performed in other joints . Elucidating the molecular mechanisms specific to post-TKA pain necessitates an animal model that replicates clinical TKA procedures, induces acute postoperative pain, and leads to complete functional recovery . Here, we present a new preclinical TKA model in rats and report on functional and behavioral outcomes indicative of pain, analgesic efficacy, serum cytokine levels, and dorsal root ganglia (DRG) transcriptomes during the acute postoperative period . Following TKA, rats exhibited marked deficits in weight bearing that persisted for 28 days . Home cage locomotion, rearing, and gait were similarly impacted and recovered by day 14 . Cytokine levels were elevated on postoperative days one and/or two . Treatment with morphine, ketorolac, or their combination improved weight bearing while gabapentin lacked efficacy . When TKA was performed in rats with OA, similar functional defi- cits and comparable recovery time courses were observed . Analysis of DRG transcriptomes revealed upregulation of transcripts linked to multiple molecular pathways including inflammation, MAPK signaling, and cytokine signaling and production . In summary, we developed a clinically relevant rat TKA model characterized by resolution of pain and functional recovery within five weeks and with pain-associated behavioral deficits that are partially alleviated by clinically administered analgesics, mirroring the postoperative experience of TKA patients .
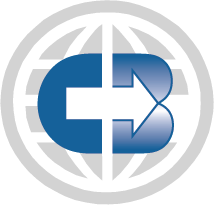

An official website of the United States government
The .gov means it’s official. Federal government websites often end in .gov or .mil. Before sharing sensitive information, make sure you’re on a federal government site.
The site is secure. The https:// ensures that you are connecting to the official website and that any information you provide is encrypted and transmitted securely.
- Publications
- Account settings
Preview improvements coming to the PMC website in October 2024. Learn More or Try it out now .
- Advanced Search
- Journal List
- J Res Med Sci
- v.17(6); 2012 Jun
Human experimental pain models: A review of standardized methods in drug development
K. sunil kumar reddy.
Department of Clinical Pharmacology and Therapeutics, ICMR Advance Centre for Clinical Pharmacodynamic, Nizam's Institute of Medical Sciences, Hyderabad, India
M. U. R. Naidu
P. usha rani, t. ramesh kumar rao.
Human experimental pain models are essential in understanding the pain mechanisms and appear to be ideally suited to test analgesic compounds. The challenge that confronts both the clinician and the scientist is to match specific treatments to different pain-generating mechanisms and hence reach a pain treatment tailored to each individual patient. Experimental pain models offer the possibility to explore the pain system under controlled settings. Standardized stimuli of different modalities (i.e., mechanical, thermal, electrical, or chemical) can be applied to the skin, muscles, and viscera for a differentiated and comprehensive assessment of various pain pathways and mechanisms. Using a multimodel-multistructure testing, the nociception arising from different body structures can be explored and modulation of specific biomarkers by new and existing analgesic drugs can be profiled. The value of human experimental pain models is to link animal and clinical pain studies, providing new possibilities for designing successful clinical trials. Spontaneous pain, the main compliant of the neuropathic patients, but currently there is no human model available that would mimic chronic pain. Therefore, current human pain models cannot replace patient studies for studying efficacy of analgesic compounds, although being helpful for proof-of-concept studies and dose finding.
INTRODUCTION
Pain is the most prevalent health care problem, and characterization of pain is of major importance in the diagnosis and choice of treatment.[ 1 ] In clinical practice, the different symptoms of the underlying disease or complaints relating to psychological, cognitive and social aspects of the illness, as well as systemic reactions such as fever and general malaise confound the characterization of pain.[ 2 ] This may bias the clinical evaluation in assessing the efficacy of analgesics; also, repeated exposures to drug make patients familiar with its side effects and increase the chance of "active placebo" effects.[ 3 ] Because of these limitations, human experimental pain models are often helpful in preclinical studies of new analgesics. Also, in human models, the investigator can control the experimentally induced pain, including the nature, localization, intensity, frequency and duration of the stimulus and provide quantitative measures of the psychological, behavioral or neurophysiological responses.[ 4 ]
Most of the animal models of pain rely on detecting a change in the threshold or response to an applied stimulus and the absence of verbal communication in animals is undoubtedly an obstacle to the evaluation of pain. Also, the neurobiology of nociceptive systems differ between species and this limits the extrapolation of findings from animal studies to man.[ 5 ] However, the animal models can be used as tools to help to find-out the relative contribution of different pain mechanisms in changing an animal's behavior in a given situation.[ 6 ]
Human experimental pain models can act as a translational bridge between animal and clinical research and many of the mechanisms tested in animals can also be translated [ Figure 1 ] and evaluated in healthy volunteers and used to predict the efficacy of a given drug in specific patient populations[ 4 ] [ Figure 2 ]. Finally, reproducibility of the method is an important factor; if reproducibility is good, the model can be useful in drug screening[ 7 ] and randomized controlled trials are the ideal to explore the effectiveness of the clinical intervention.[ 8 ]

Human experimental pain models: Focus on translation

Examples for the scope of pain indications
Assessment of the output from these pain models can be based on psychophysical or neurophysiological methods.[ 9 ] Psychophysical methods are the simplest way to assess the pain response[ 10 ] and are based on subjective experience of pain, measured on standard scales or as pain thresholds and neurophysiological methods include measurement of evoked brain potentials or nociceptive withdrawal reflexes.
DEFINITIONS
Pain due to a stimulus which does not normally provoke pain [ Figure 3 ].

Illustrates hyperalgesia; manifested locally (primary hyperalgesia) and by central sensitization (secondary hyperalgesia), for example, after freeze injury or 30 minutes after application of capsaicin
Hyperalgesia
An increased response to a noxious stimulus, caused by sensitization of peripheral nociceptors (primary hyperalgesia: The area of tissue injury) and/or by sensitization of central neurons (secondary hyperalgesia: outside the area of the original tissue injury) [ Figure 3 ].
Temporal Summation/Windup Like Pain
It is a phenomenon that occurs when a repetition of a stimulus increases pain perception.
Spatial summation
It is a phenomenon that occurs when a non-painful stimulus is perceived as painful when applied to a wider area.
Referred pain
Pain that is felt in a part of the body at a distance from the area of stimulation.
PAIN-INDUCTION METHODS
Pain models can be classified according to their presumed mechanism (inflammatory vs neuropathic), the involved tissue (skin vs muscle vs viscera) and their time course (phasic - shorter, lasting for milliseconds to seconds vs tonic - longer, lasting for minutes). However, mechanistically the most important categories are peripheral or central sensitization. Conceptually, the two forms of sensitization are strictly separated; but, most of the pain models are characterized by a combination of peripheral and central sensitization. For example, cutaneous freeze injury lead to both allodynia and hyperalgesia.[ 11 ] The present review gives a brief overview of the pain models according to the tissue type, with an intention for the development of the sensitive pain model methods based on the knowledge of earlier methods described in the present review.
MECHANICAL STIMULATION
Mechanical stimulation of the skin.
Mechanical stimulation of the skin can be grouped into Touch, Pin Prick, and Pinching. This method has been used to evaluate the effect of clonidine, oxycodone, morphine, mepivacain, bupivacain, gabapentin, carbamazepine, and amitriptyline.
Sensitivity to touch can be assessed by applying a light pressure with a finger[ 12 ] or using a von Frey hair.[ 13 ] A-beta fiber mediate touch sensation.[ 14 ]
Major shortcomings
The von Frey hair activates both low threshold mechanoreceptors and nociceptors and is not specific.[ 6 ] Also, touch is used mainly to explore allodynia evoked by other pain stimuli.[ 15 ]
Stimulation of the skin gently with a needle[ 16 ] or a safety pin[ 17 ] or thick von Frey filament and pain is reported as pricking or "first pain".[ 15 ] Pin-prick stimulation predominantly activates A-delta fibres.[ 14 ]
The rapidity of pain onset and termination is not easily controlled.[ 6 , 18 ]
A skin flap can be squeezed between two pressure sensors[ 19 ] or a finger, toe[ 20 ] or an ear lobe[ 16 ] can be pinched between the algometer probe and a pinch handle.
The rapidity of pain onset and termination is not easily controlled.[ 6 , 18 ] Pain during pinching is a combination of mechanical stimulation and local ischemia.[ 21 ]
Mechanical stimulation of the muscle
This is typical exogenous experimental pain model and has been used for drug assessment of morphine, oxycodone, rofecoxib, tramadol, codeine, imipramine, and ketamine.
Pressure algometry is the most frequently applied technique for quantification of pain, where the probe is applied to a hard body structures, such as periosteum[ 16 ] or soft tissue such as muscles.[ 19 ] Both A-delta and C-fibers mediate pain induced by pressure stimulation.[ 22 ]
The technique is nonspecific since receptors in the skin, and probably deeper tissues will be activated.
Mechanical stimulation of the viscera
Mechanical stimulation of viscera has been used to evaluate the effect of serotonin (5-HT) 4 receptor agonist tegaserod, as well as oxycodone, morphine, Octreotide (a somatostatin analog), nifedipine, NMDA receptor antagonist ketamine, tricyclic antidepressants like imipramine, amitriptyline.
Mechanical stimulation in viscera using balloon distension has been widely used to study pain perception thresholds, referred pain and cerebral activation patterns[ 23 ] as well as, to screen new analgesics in healthy subjects and those with gut disorders.[ 24 , 25 ] The introduction of the electronic barostat, has helped to ensure proper location of the balloon, regardless of the inflation paradigm that was used. The newer methods based on impedance planimetry, allows recording of the luminal cross-sectional area directly and estimation of the radius in the distended segment of the esophagus or intestine.[ 26 ]
Unlike the rectum and the stomach, the esophagus doesn′t serve as a storage organ, but rather as a conduct. Consequently intraesophageal distensions do not mimic a normal, physiologic stimulus and thus perceptual responses to such a stimulus may have no scientific merit. In addition, difficulties in tolerating balloon distension, commonly results in poor recruitment rates as well as the potential for esophageal perforation, have made esophageal balloon distensions by a barostat a less attractive research tool.
THERMAL STIMULATION
Thermal stimulation of the skin.
A cold stimulation/cold pressor pain . Application of ice,[ 27 ] a cold gel bag,[ 28 ] a wet alcohol sponge[ 12 ] menthol[ 29 , 30 ] ether, or a Peltier thermode to the skin evokes cold sensation. The method has been intensively used for drug screening such as imipramine, paroxetine, morphine, codeine, tramadol, and oxycodone.
Nociceptors of cutaneous veins appear to mediate cold pain in humans[ 31 ] via activation of A-delta and C-fibres.[ 32 ] However, a lack of standardization in the cold pressor model is revealed, with substantial variation in both equipment and methodology used.[ 33 ]
Vascular reactions strongly affect the response and cold pressor pain has shown contradictionary results in the testing of analgesics.[ 7 ] Also, pain onset and offset are gradual, and the experiment is not repeatable within a 1--2 hour session.[ 18 ]

Freeze lesion
Application of cold temperatures (-20°C) at a standardised pressure provides stable testing conditions for 1 day.[ 34 ]
A central component of the analgesia can be explored, even if the method is thought to evoke mainly peripheral mechanisms.[ 35 ]
Contact heat
The most commonly used heat/cold contact stimulators are based on the Peltier principle (one direction of the current causes cooling and the other way heating).[ 36 ] Warm sensation is mediated by C-fibers.[ 37 , 38 ] At threshold determinations, rapid skin heating activates first A-delta fibres, corresponds to the "first pain" followed by a C-fibre mediated second pain, being described as ′throbbing, burning or swelling′.[ 39 ] Slow heating gives a preferential activation of C-fibers (thought to be most important for peripheral opioid receptors) and the best evaluation of second pain.[ 7 ] In order to prevent injuries to the skin, the high temperature limit is recommended to be 50 °C and the low limit 5 °C.[ 40 ]
Contact of the thermode with the skin activates concomitant low-threshold non-nociceptors. The rate of thermal transfer depends on the thermode-skin contact and thus on the pressure of application of the thermode. Therefore it is important that the thermode is applied to skin at a standardized fashion.
Radiant heat
Laser pulses evoke a distinct pricking pain in skin.[ 40 , 41 ] Intensities higher than those evoking pricking pain are avoided, as they may cause superficial burns.[ 42 ] Depending on the stimulus intensity, laser-induced thermal stimulation produces a double pain sensation: an initial prick sensation attributable to A-delta fiber activity[ 41 ] and a second diffuse burning sensation due to C-fiber activity.[ 43 ] Different laser emission sources have been developed in pain research e.g. argon, copper vapor[ 44 , 45 ] thulium-YAG and laser diodes, but CO 2 -laser[ 46 ] is most commonly used.[ 40 ] The argon[ 44 ] and copper vapor lasers[ 45 ] operate, a different principle than CO 2 -laser, where in CO 2 -laser, radiation is absorbed within the epidermis, independent of skin pigmentation and application angle. Whereas, in the Argon-laser much of the radiant heat is reflected, causing variation depending on the skin pigmentation and the application angle.[ 47 ] In all laser studies, the spot of stimulation has to be shifted slightly between consecutive stimuli in order not to cause receptor sensitization/fatigue. Irradiation using ultraviolet B (UVB), produces stable areas of primary hyperalgesia over several hours.[ 48 , 49 ]
Variability in responses between the individuals was observed.[ 6 , 47 ]
Burn injury
The burn injury model illustrates hyperalgesia. Contact heat and radiant heat can induce burn injury by, e.g., application of a constant temperature of 47 °C for 5 min (leads to long lasting sensitization). The brief thermal sensitization model (BTS; 45 °C for 3 min) provides short lasting sensitization and can be induced 2-3 times at hourly intervals without skin injury. Among the endogenous inflammatory mediators, prostaglandin E2 may be responsible for early heat hyperalgesia.[ 50 ] Whereas in longer lasting mechanical hyperalgesia nerve growth factor may be involved.[ 51 , 52 ] Both A-delta and C-fibres mediate pain after a burn injury.[ 15 ]
The threshold for activation of mainly A delta-fibres may transform into thresholds for activation of C-fibres. As these respond to other stimulus modalities it may confuse the testing of an analgesic.[ 6 ]
Thermal stimulation of the muscle
Warm and cold pain has been evoked from muscle tissue when saline at different temperatures is injected intramuscularly.[ 53 ]
No drug studies have been performed.
Thermal stimulation of viscera
Phasic thermal stimuli of the human gastrointestinal tract are believed to activate unmyelinated afferents in the mucosa selectively. This is opposed to mechanical and electrical stimuli, which activate afferents in both superficial and deep layers.[ 54 ] Thermosensitive mucosal afferents have been demonstrated in the human esophagus, stomach and rectum.[ 26 , 54 ] Human gastrointestinal tract shows a uniform perception of thermal stimuli with different reflex responses from the stomach to the jejunum.[ 55 ] The temperature of recirculating water, when continuously measured inside a balloon positioned in the esophagus, showed a linear stimulus-response relationship, demonstrating the validity of the activation.[ 26 ]
Major shortcomings: Only a few pharmacological studies have been performed using thermal visceral stimulation (e.g., oxycodone and morphine).
ELECTRICAL STIMULATION
Electrical stimulation of the skin.
Various electrical stimulator devices connected to electrodes applied either to the skin surface[ 28 ] or the intracutaneous tissue[ 56 ] evoke electrical stimulation. Stimulator devices can deliver different stimulation pattern, for example, different waveforms, frequencies and duration of stimuli. This activates with some selectivity different afferents and nervous structures, and hence evokes different kinds of pain.[ 6 , 7 ] Electrical stimulation excites nerve fiber populations and the relative proportion of activation of individual fiber types depends on the stimulus intensity.[ 7 ] C-fibers have a higher activation threshold than A-delta fibers. Drug studies on drug classes like opioids, tricyclic antidepressants and NSAIDs have been performed using electrical stimulus of different intensities.
Electrical stimulation bypasses the receptors and activates the nerve fibers directly, and the method is not a specific activation of the nociceptors. The electrical threshold is related to the fiber diameter and one cannot usually excite small-diameter nerves without additionally exciting others.
Electrical stimulation of the muscle
Electrical stimulation of the muscle can be performed via small needle electrodes with un-insulated tips.[ 57 ] Repeated electrical stimulation can induce temporal summation and cause increase in referred pain areas, thus reflecting central changes.[ 58 , 59 ] The technique has been used to evaluate drugs such as remifentanil, morphine, alfentanil, oxycodone, and ketamine.
Electrical stimulation is not nociceptive specific as it bypasses the receptors. Furthermore, concurrent activated muscle twitches may confound the sensation evoked by intramuscular electrical stimulation.[ 60 ]
Electrical stimulation of the viscera
Electrical stimulation of the gut has been widely used to study basic pain mechanisms, pain characteristics, referred pain and evoked brain potentials. The use of electrical stimulation has demonstrated safety in all parts of the gastrointestinal system. Furthermore, the well-defined onset and offset of the stimulation eliminates the latency as observed with other methods, making this particularly suitable for neurophysiological assessments.-[ 2 , 61 ] The technique has been used successfully in drug studies of valdecoxib, parecoxib, morphine, oxycodone, and ketamine.
Electrical gut stimulation, bypasses the receptors and activates the nerve fibers directly. The major drawback with earlier methods was the varying electrode contact with the mucosa, giving inconsistent results.
CHEMICAL STIMULATION
Chemical stimulation of the skin.
Intradermal injection or topical application of capsaicin directly evokes pain, and hyperalgesia.[ 62 ] Capsaicin induced pain has been suggested as a surrogate model of changes observed in neuropathic pain. Mostly C-fibres are thought to mediate pain.[ 63 ] Capsaicin application activates t ransient receptor potential vanilloid 1 (TRPV1) receptors. This model is widely used for drug evaluations, such as the effect of neurotoxins (e.g., Botox) on TRPV1 sensitive nociceptive endings, as well as ketamine, magnesium, lidocaine, alfentanil, diclofenac, orphenadrine, gabapentin, cannabis, lamotrigine, H 1 antagonists, hydromorphone, and the lidocaine patch.
Variable response was obtained with the model, e.g., pharmacological testing of lamotrigine and desipramine which are used in the treatment of neuropathic pain failed to show any effects in the model.[ 63 , 64 ] On the other hand gabapentin, which is also used to treat neuropathic pain, suppresses hyperalgesia following heat-capsaicin sensitization.[ 65 ]
Mustard oil
Topical application of mustard oil induces pain and hyperalgesia. C-fibres are thought to mediate the burning pain, while A-beta fibers are believed to mediate allodynia to light mechanical stimuli.[ 15 ]
The method has not been used much in the testing of analgesics. The use of these models is basically limited to target engagement studies.
Chemical stimulation of muscle
Intramuscular infusion of hypertonic saline[ 59 , 66 ] glutamate[ 67 ] and capsaicin[ 68 ] induces pain and referred pain areas. Hypertonic saline mimics musculoskeletal pain in both subjectively perceived quality as well as its effects on motor performance.[ 69 ] The dominant sensation following hypertonic saline injections in the muscle is a deep and diffuse pain, via activation of C-fibres. Earlier manual bolus infusions of hypertonic saline were used. Standardization of a small bolus volume is easy to accomplish by a computer-controlled infusion pump. This provides more reproducible method[ 60 ] and have been used to evaluate the effects of the NMDA receptor blocker, ketamine, as well as morphine and alfentanil. Intramuscular injections of algesic substances such as bradykinin[ 70 ] , serotonin[ 70 ] , substance P[ 70 ] , potassium chloride, L-ascorbic acid, and acid phosphate buffer are other chemical stimulation methods to evoke muscular pain.
Hypertonic saline injections may excite both non-nociceptive and nociceptive nerve fibers. The chemical stimulation methods all have a problematic reproducibility with large interindividual differences.[ 71 ]
Chemical stimulation of the viscera
Chemical stimulation is believed to be the ideal experimental visceral pain stimulus, closely resembles clinical inflammation. Acid stimulation of the esophagus is the most common method to sensitize the gut.[ 72 ] Application of glycerol to the large intestine evoked pain in patients with the irritable bowel syndrome.[ 73 ] In the colon mucosa, injections of 2%--6% hypertonic saline resulted in deep as well as referred nonpainful and painful perceptions.[ 2 ] Most chemical stimuli are believed to activate predominantly unmyelinated C-fibers.[ 74 ] Application of capsaicin in the ileum resulted in a dose-dependent pain response and referred pain.[ 2 ] Chemical stimulation of gut using algogenic substances like alcohol, bradykinin has also been performed in humans.[ 2 ]
The major disadvantage of chemical stimulation is a relatively long latency time to the onset of effects and often responses are not reproducible when repeated.
ENDOGENOUS METHODS OF MUSCLE STIMULATION
Ischemic stimulation.
The tourniquet model is a classical experimental pain model that induces ischemic muscle pain. Earlier methods of producing experimental pain by occluding the blood flow of exercising muscles failed to demonstrate satisfactory response. The modified method developed by smith et al .[ 75 ] more closely resembles pain of pathologic origin. It has been used in human analgesic assays such as morphine, tramadol, caffeine, rofecoxib, aspirin, ibuprofen. This model is applicable in experimental studies requiring a general tonic pain stimulus.[ 60 ]
It is a very efficient model to induce pain in the muscles but is non-specific, since skin, periosteum, and other tissues will contribute to the overall pain perception. When activating nociceptors, concomitantly low-threshold non-nociceptive nerves can be activated by the contact of the tourniquet with the skin. This activation can exert an inhibitory influence on pain mechanisms.
Pain evoked by exercise
Delayed onset muscle soreness (DOMS) is a sensation of muscular pain during active contractions or passive stretch of a muscle after unaccustomed or eccentric exercise, which peaks 24-48 hours after exercise.[ 76 ] DOMS is thought to be caused by structural damage to muscle that leads to the release in the muscle of algogenic substances such as prostaglandins.[ 77 – 79 ] These algogenic substances sensitize A-delta and C nociceptive fibres.[ 79 ] Large mechanoreceptor afferents from muscle, muscle spindle and tendons are activated in DOMS, and may also contribute to the pain syndromes.[ 80 – 84 ] This model was used for drug evaluations, such as morphine, tramadol, codeine, ketoprofen, diclofenac, ibuprofen, rofecoxib and naproxen.
Neural mechanisms that leads to DOMS were incompletely understood. Variable results were obtained.[ 85 , 86 , 87 ] Animal studies show that a stress-induced analgesia can occur with eccentric exercise.[ 88 ] These also bias the results in analgesic testing.
SCOPE OF TRANSLATIONAL PAIN RESEARCH
Traditionally, translational research is regarded as a process of bridging bench findings to clinical application and the process requires coordinated bidirectional approaches between bedside and bench because of the subjective nature of the pain. This is an advantage in proof-of-concept studies, where the efficacy of a given compound on specific mechanisms can be assessed and the dose-response relationship can be determined.
The link between sensitization in inflammatory models such as UVB burn and clinical inflammatory conditions is considerably good. Also, a new model of peripheral and central sensitization without inflammation: intracutaneous injection of nerve growth factor[ 89 ] which appears to generate a combination of symptoms similarly found in patients. However, even these models only reflect part of the disease. Still we lack information about the role of trophic factors for the long-term modulation of nociceptor structure and sensitivity. Therefore, current theories or concepts of pain mechanisms need to be critically reviewed and analyzed to provide a new roadmap of contemporary pain research.
Spontaneous pain is a salient feature of clinical pain, which is the main complaint of neuropathic patients, reported to have more severe depression and physical disability[ 90 ] is not mimicked in most of the human pain models.[ 10 ] This essential gap is based on ethical limitations of human models according to which no healthy volunteers can be turned into a chronic pain patient. Irrespective of this limitation, the mechanism leading to spontaneous pain is unknown and even the site of origin is debated.
Finally, the pain arising from the skin, muscles and viscera differ from one another, and hence compounds may show very different effects on pain from these structures. Therefore, the concept of multimodel, multitissue pain assessment has been developed, where advanced drug screening in healthy volunteers and patients is possible. Therefore, a meaningful translation process through clinical studies should begin with careful choices of appropriate clinical pain conditions that are consistent with the conditions examined in preclinical models.
ACKNOWLEDGMENTS
We thank the Director, Nizam's Institute of Medical Sciences for providing necessary infrastructure.
Source of Support: ICMR, Government of India
Conflict of Interest: None declared.
Animal Models of Inflammatory Pain
- First Online: 01 January 2010
Cite this protocol
- Rui-Xin Zhang 3 &
Part of the book series: Neuromethods ((NM,volume 49))
1566 Accesses
9 Citations
Animal models of inflammatory pain have been widely used to study the mechanisms of tissue injury-induced persistent pain. A variety of inflammatory agents or irritants, including complete Freund’s adjuvant, carrageenan, zymosan, mustard oil, formalin, capsaicin, bee venom, acidic saline, lipopolysaccharide, inflammatory cytokines, and sodium urate crystals, have been used to produce tissue injury and hyperalgesia in such structures as cutaneous/subcutaneous tissues, joints, and muscles. Additionally, models of pain hypersensitivity have also been established with injuries produced by burning, freezing, and ultra irradiation. Although these models do not simulate every aspect of chronic pain, they do model key features of human inflammatory pain. Studies in animals give insight into certain aspects of human pain conditions and lead to improved pain management for patients.
This is a preview of subscription content, log in via an institution to check access.
Access this chapter
Subscribe and save.
- Get 10 units per month
- Download Article/Chapter or eBook
- 1 Unit = 1 Article or 1 Chapter
- Cancel anytime
- Available as PDF
- Read on any device
- Instant download
- Own it forever
- Available as EPUB and PDF
- Compact, lightweight edition
- Dispatched in 3 to 5 business days
- Free shipping worldwide - see info
- Durable hardcover edition
Tax calculation will be finalised at checkout
Purchases are for personal use only
Institutional subscriptions
Similar content being viewed by others
Animal Models of Pain and Anti-inflammatory Treatments
Animal models of rheumatoid pain: experimental systems and insights, inflammation and pain.
Bouncier, M., Cavey, D., Kail, N., and Hensby, C. (1990) Experimental models in skin pharmacology, Pharmacological Reviews 42 , 127–154.
Google Scholar
Le Bars, D., Gozariu, M., and Cadden, S. W. (2001) Animal models of nociception, Pharmacological Reviews 53 , 597–652.
PubMed Google Scholar
Colpaert, F. C. (1987) Evidence that adjuvant arthritis in the rat is associated with chronic pain, Pain 28 , 201–222.
Article PubMed CAS Google Scholar
Millan, M. J., Czlonkowski, A., Morris, B., Stein, C., Arendt, R., Huber, A., Hollt, V., and Herz, A. (1988) Inflammation of the hind limb as a model of unilateral, localized pain: influence on multiple opioid systems in the spinal cord of the rat, Pain 35 , 299–312.
Iadarola, M. J., Brady, L. S., Draisci, G., and Dubner, R. (1988) Enhancement of dynorphin gene expression in spinal cord following experimental inflammation: stimulus specificity, behavioral parameters and opioid receptor binding, Pain 35 , 313–326.
Zhang, R.-X., Chen, J. Y., Ruda, M. A., and Qiao, J.-T. (1999) Functional relationship between peripheral inflammation, thermal hyperalgesia, and enhanced dynorphin gene expression in spinal cord of rat: behavioral and mRNA studies, Chinese Journal of Neuro anatomy 15 , 19–26.
Lao, L., Zhang, R.-X., Zhang, G., Wang, X., Berman, B. M., and Ren, K. (2004) A parametric study of electroacupuncture on persistent hyperalgesia and Fos protein expression in rats, Brain Research 1020 , 18–29.
Gould, H. J. III. (2000) Complete Freund’s adjuvant-induced hyperalgesia: a human perception, Pain 85 , 301–303.
Article PubMed Google Scholar
Zhang, R.-X., Lao, L., Qiao, J.-T., and Ruda, M. A. (2003) Strain differences in pain sensitivity and expression of preprodynorphin mRNA in rats following peripheral inflammation, Neuroscience Letters 353 , 213–216.
Vinegar, R., Schreiber, W., and Hugo, R. (1969) Biphasic development of carrageenin edema in rats, Journal of Pharmacology and Experimental Therapeutics 166 , 96–103.
PubMed CAS Google Scholar
Winter, C. A., Risley, E. A., and Nuss, G. W. (1962) Carrageenin-induced edema in hind paw of the rat as an assay for antiiflammatory drugs, Proceedings of the Society for Experimental Biology and Medicine 111 , 544–547.
Fecho, K., Nackley, A. G., Wu, Y., and Maixner, W. (2005) Basal and carrageenan-induced pain behavior in Sprague-Dawley, Lewis and Fischer rats, Physiology & Behavior 85 , 177–186.
Article CAS Google Scholar
Yeo, J. F., Ong, W. Y., Ling, S. F., and Farooqui, A. A. (2004) Intracerebroventricular injection of phospholipases A2 inhibitors modulates allodynia after facial carrageenan injection in mice, Pain 112 , 148–155.
Ng, C. H. and Ong, W. Y. (2002) Increased synaptosomal [3H] GABA uptake in the rat brainstem after facial carrageenan injections, Pain 98 , 259–268.
Imbe, H., Iwata, K., Zhou, Q. Q., Zou, S., Dubner, R., and Ren, K. (2001) Orofacial deep and cutaneous tissue inflammation and trigeminal neuronal activation. Implications for persistent temporomandibular pain, Cells Tissues Organs 169 , 238–247.
Bautista, D. M., Jordt, S. E., Nikai, T., Tsuruda, P. R., Read, A. J., Poblete, J., Yamoah, E. N., Basbaum, A. I., and Julius, D. (2006) TRPA1 mediates the inflammatory actions of environmental irritants and proalgesic agents, Cell 124 , 1269–1282.
Inoue, H., Asaka, T., Nagata, N., and Koshihara, Y. (1997) Mechanism of mustard oil-induced skin inflammation in mice, European Journal of Pharmacology 333 , 231–240.
Lippe, I. T., Stabentheiner, A., and Holzer, P. (1993) Participation of nitric oxide in the mustard oil-induced neurogenic inflammation of the rat paw skin, European Journal of Pharmacology 232 , 113–120.
Urban, M. O., Jiang, M. C., and Gebhart, G. F. (1996) Participation of central descending nociceptive facilitatory systems in secondary hyperalgesia produced by mustard oil, Brain Research 737 , 83–91.
Meller, S. T. and Gebhart, G. F. (1997) Intraplantar zymosan as a reliable, quantifiable model of thermal and mechanical hyperalgesia in the rat, European Journal of Pain 1 , 43–52.
Dubuisson, D. and Dennis, S. G. (1977) The formalin test: a quantitative study of the analgesic effects of morphine, meperidine, and brain stem stimulation in rats and cats, Pain 4 , 161–174.
Abbott, F. V., Franklin, K. B. J., and Westbrook, R. F. (1995) The formalin test: scoring properties of the first and second phases of the pain response in rats, Pain 60 , 91–102.
Puig, S. and Sorkin, L. S. (1996) Formalin-evoked activity in identified primary afferent fibers: systemic lidocaine suppresses phase-2 activity, Pain 64 , 345–355.
Pitcher, G. M. and Henry, J. L. (2002) Second phase of formalin-induced excitation of spinal dorsal horn neurons in spinalized rats is reversed by sciatic nerve block, European Journal of Neuroscience 15 , 1509–1515.
Taylor, B. K., Peterson, M. A., and Basbaum, A. I. (1995) Persistent cardiovascular and behavioral nociceptive responses to subcutaneous formalin require peripheral nerve input, Journal of Neuroscience 15 , 7575–7584.
Abbadie, C., Taylor, B. K., Peterson, M. A., and Basbaum, A. I. (1997) Differential contribution of the two phases of the formalin test to the pattern of c-fos expression in the rat spinal cord: studies with remifentanil and lidocaine, Pain 69 , 101–110.
Henry, J. L., Yashpal, K., Pitcher, G. M., and Coderre, T. J. (1999) Physiological evidence that the ‘interphase’ in the formalin test is due to active inhibition, Pain 82 , 57–63.
Johansen, J. P., Fields, H. L., and Manning, B. H. (2001) The affective component of pain in rodents: direct evidence for a contribution of the anterior cingulate cortex, Proceedings of the National Academy of Sciences of the United States of America 98 , 8077–8082.
Raboisson, P. and Dallel, R. (2004) The orofacial formalin test, Neuroscience and Biobehavioral Reviews 28 , 219–226.
Yeo, J. F. (2002) Does nitric oxide play a role in orofacial pain transmission? Annals of the New York Academy of Sciences 962 , 151–160.
Clavelou, P., Dallel, R., Orliaguet, T., Woda, A., and Raboisson, P. (1995) The orofacial formalin test in rats: effects of different formalin concentrations, Pain 62 , 295–301.
Chen, J., Luo, C., Li, H.-L., and Chen, H.-S. (1999) Primary hyperalgesia to mechanical and heat stimuli following subcutaneous bee venom injection into the plantar surface of hindpaw in the conscious rat: a comparative study with the formalin test, Pain 83 , 67–76.
LaMotte, R. H., Shain, C. N., Simone, D. A., and Tsai, E. F. (1991) Neurogenic hyperalgesia: psychophysical studies of underlying mechanisms, Journal of Neurophysiology 66 , 190–211.
Sumikura, H., Andersen, O. K., Drewes, A. M., and Arendt-Nielsen, L. (2003) Spatial and temporal profiles of flare and hyperalgesia after intradermal capsaicin, Pain 105 , 285–291.
Serra, J., Campero, M., and Ochoa, J. (1998) Flare and hyperalgesia after intradermal capsaicin injection in human skin, Journal of Neurophysiol 80 , 2801–2810.
CAS Google Scholar
Scanlon, G. C., Wallace, M. S., Ispirescu, J. S., and Schulteis, G. (2006) Intradermal capsaicin causes dose-dependent pain, allodynia, and hyperalgesia in humans, Journal of Investigative Medicine 54 , 238–244.
Gazerani, P., Andersen, O. K., and Arendt-Nielsen, L. (2007) Site-specific, dose-dependent, and sex-related responses to the experimental pain model induced by intradermal injection of capsaicin to the foreheads and forearms of healthy humans, Journal of Orofacial Pain 21 , 289–302.
Dougherty, P. M., Schwartz, A., and Lenz, F. A. (1999) Responses of primate spinomesencephalic tract cells to intradermal capsaicin, Neuroscience 90 , 1377–1392.
Gilchrist, H. D., Allard, B. L., and Simone, D. A. (1996) Enhanced withdrawal responses to heat and mechanical stimuli following intraplantar injection of capsaicin in rats, Pain 67 , 179–188.
Alvarez, P., Saavedra, G., Hernandez, A., Paeile, C., and Pelissier, T. (2003) Synergistic antinociceptive effects of ketamine and morphine in the orofacial capsaicin test in the rat, Anesthesiology 99 , 969–975.
Pelissier, T., Pajot, J., and Dallel, R. (2002) The orofacial capsaicin test in rats: effects of different capsaicin concentrations and morphine, Pain 96 , 81–87.
Chen, H.-S., He, X., Wang, Y., Wen, W.-W., You, H.-J., and Arendt-Nielsen, L. (2007) Roles of capsaicin-sensitive primary afferents in differential rat models of inflammatory pain: a systematic comparative study in conscious rats, Experimental Neurology 204 , 244–251.
De Castro Costa, M., De Sutter, P., Gybels, J., and Van Hees, J. (1981) Adjuvant-induced arthritis in rats: a possible animal model of chronic pain, Pain 10 , 173–185.
Millan, M. J., Czlonkowski, A., Pilcher, C. W., Almeida, O. F., Millan, M. H., Colpaert, F. C., and Herz, A. (1987) A model of chronic pain in the rat: functional correlates of alterations in the activity of opioid systems, Journal of Neuroscience 7 , 77–87.
Coderre, T. J. and Wall, P. D. (1987) Ankle joint urate arthritis (AJUA) in rats: an alternative animal model of arthritis to that produced by Freund’s adjuvant, Pain 28 , 379–393.
Baek, Y. H., Choi, D. Y., Yang, H. I., and Park, D. S. (2005) Analgesic effect of electroacupuncture on inflammatory pain in the rat model of collagen-induced arthritis: mediation by cholinergic and serotonergic receptors, Brain Research 1057 , 181–185.
Wu, J., Lin, Q., Lu, Y., Willis, W. D., and Westlund, K. N. (1998) Changes in nitric oxide synthase isoforms in the spinal cord of rat following induction of chronic arthritis, Experimental Brain Research 118 , 457–465.
Barton, N. J., Strickland, I. T., Bond, S. M., Brash, H. M., Bate, S. T., Wilson, A. W., Chessell, I. P., Reeve, A. J., and McQueen, D. S. (2007) Pressure application measurement (PAM): a novel behavioural technique for measuring hypersensitivity in a rat model of joint pain, Journal of Neuroscience Methods 163 , 67–75.
Butler, S. H., Godefroy, F., Besson, J. M., and Weil-Fugazza, J. (1992) A limited arthritic model for chronic pain studies in the rat, Pain 48 , 73–81.
Sluka, K. A., Bailey, K., Bogush, J., Olson, R., and Ricketts, A. (1998) Treatment with either high or low frequency TENS reduces the secondary hyperalgesia observed after injection of kaolin and carrageenan into the knee joint, Pain 77 , 97–102.
Sluka, K. A. and Westlund, K. N. (1993) Behavioral and immunohistochemical changes in an experimental arthritis model in rats, Pain 55 , 367–377.
Ji, G., Fu, Y., Ruppert, K. A., and Neugebauer, V. (2007) Pain-related anxiety-like behavior requires CRF1 receptors in the amygdala, Molecular Pain 3 , 13.
Schaible, H. G. and Schmidt, R. F. (1985) Effects of an experimental arthritis on the sensory properties of fine articular afferent units, Journal of Neurophysiology 54 , 1109–1122.
Dougherty, P. M., Sluka, K. A., Sorkin, L. S., Westlund, K. N., and Willis, W. D. (1992) Neural changes in acute arthritis in monkeys. I. Parallel enhancement of responses of spinothalamic tract neurons to mechanical stimulation and excitatory amino acids, Brain Research. Brain Research Reviews 17 , 1–13.
Radhakrishnan, R., Moore, S. A., and Sluka, K. A. (2003) Unilateral carrageenan injection into muscle or joint induces chronic bilateral hyperalgesia in rats, Pain 104 , 567–577.
Martins, M. A., De Castro Bastos, L., and Tonussi, C. R. (2006) Formalin injection into knee joints of rats: pharmacologic characterization of a deep somatic nociceptive model, Journal of Pain 7 , 100–107.
Roveroni, R. C., Parada, C. A., Cecília, M., Veiga, F. A., and Tambeli, C. H. (2001) Development of a behavioral model of TMJ pain in rats: the TMJ formalin test, Pain 94 , 185–191.
Okuda, K., Nakahama, H., Miyakawa, H., and Shima, K. (1984) Arthritis induced in cat by sodium urate: a possible animal model for tonic pain, Pain 18 , 287–297.
Sluka, K. A. and Wright, A. (2001) Knee joint mobilization reduces secondary mechanical hyperalgesia induced by capsaicin injection into the ankle joint, European Journal of Pain 5 , 81–87.
Lam, F. Y. and Ferrell, W. R. (1991) Neurogenic component of different models of acute inflammation in the rat knee joint, Annals of the Rheumatic Diseases 50 , 747–751.
Reeh, P. W. and Steen, K. H. (1996) Tissue acidosis in nociception and pain, Progress in Brain Research 113 , 143–151.
Steen, K. H., Reeh, P. W., Anton, F., and Handwerker, H. O. (1992) Protons selectively induce lasting excitation and sensitization to mechanical stimulation of nociceptors in rat skin, in vitro, Journal of Neuroscience 12 , 86–95.
Sluka, K. A., Kalra, A., and Moore, S. A. (2001) Unilateral intramuscular injections of acidic saline produce a bilateral, long-lasting hyperalgesia, Muscle & Nerve 24 , 37–46.
Ledeboer, A., Mahoney, J. H., Milligan, E. D., Martin, D., Maier, S. F., and Watkins, L. R. (2006) Spinal cord glia and interleukin-1 do not appear to mediate persistent allodynia induced by intramuscular acidic saline in rats, Journal of Pain 7 , 757–767.
Diehl, B., Hoheisel, U., and Mense, S. (1988) Histological and neurophysiological changes induced by carrageenan in skeletal muscle of cat and rat, Agents and Actions 25 , 210–213.
Kehl, L. J., Trempe, T. M., and Hargreaves, K. M. (2000) A new animal model for assessing mechanisms and management of muscle hyperalgesia, Pain 85 , 333–343.
Beyreuther, B. K., Geis, C., Stöhr, T., and Sommer, C. (2007) Antihyperalgesic efficacy of lacosamide in a rat model for muscle pain induced by TNF, Neuropharmacology 52 , 1312–1317.
Dina, O. A., Green, P. G., and Levine, J. D. (2008) Role of interleukin-6 in chronic muscle hyperalgesic priming, Neuroscience 152 , 521–525.
Ro, J. Y., Capra, N., and Masri, R. (2003) Development of a behavioral assessment of craniofacial muscle pain in lightly anesthetized rats, Pain 104 , 179–185.
Ro, J. Y. (2005) Bite force measurement in awake rats: a behavioral model for persistent orofacial muscle pain and hyperalgesia, Journal of Orofacial Pain 19 , 159–167.
Nozaki-Taguchi, N. and Yaksh, T. L. (1998) A novel model of primary and secondary hyperalgesia after mild thermal injury in the rat, Neuroscience Letters 254 , 25–28.
Wang, S., Lim, G., Yang, L., Zeng, Q., Sung, B., Jeevendra Martyn, J. A., and Mao, J. (2005) A rat model of unilateral hindpaw burn injury: Slowly developing rightwards shift of the morphine dose-response curve, Pain 116 , 87–95.
Hylden, J. L., Thomas, D. A., Iadarola, M. J., Nahin, R. L., and Dubner, R. (1991) Spinal opioid analgesic effects are enhanced in a model of unilateral inflammation/hyperalgesia: possible involvement of noradrenergic mechanisms, European Journal of Pharmacology 194 , 135–143.
Pedersen, J. L. and Kehlet, H. (1998) Secondary hyperalgesia to heat stimuli after burn injury in man, Pain 76 , 377–384.
Chassaing, C., Schmidt, J., Eschalier, A., Cardot, J. M., and Dubray, C. (2006) Hyperalgesia induced by cutaneous freeze injury for testing analgesics in healthy volunteers, British Journal of Clinical Pharmacology 61 , 389–397.
Bishop, T., Hewson, D. W., Yip, P. K., Fahey, M. S., Dawbarn, D., Young, A. R., and McMahon, S. B. (2007) Characterisation of ultraviolet-B-induced inflammation as a model of hyperalgesia in the rat, Pain 131 , 70–82.
Harrison, G. I., Young, A. R., and McMahon, S. B. (2004) Ultraviolet radiation-induced inflammation as a model for cutaneous hyperalgesia, Journal of Investigative Dermatology 122 , 183–189.
Chacur, M., Longo, I., Picolo, G., Gutiérrez, J. M., Lomonte, B., Guerra, J. L., Teixeira, C. F. P., and Cury, Y. (2003) Hyperalgesia induced by Asp49 and Lys49 phospholipases A2 from Bothrops asper snake venom: pharmacological mediation and molecular determinants, Toxicon 41 , 667–678.
Maier, S. F., Wiertelak, E. P., Martin, D., and Watkins, L. R. (1993) Interleukin-1 mediates the behavioral hyperalgesia produced by lithium chloride and endotoxin, Brain Research 623 , 321–324.
Watkins, L. R., Wiertelak, E. P., Goehler, L. E., Mooney-Heiberger, K., Martinez, J., Furness, L., Smith, K. P., and Maier, S. F. (1994) Neurocircuitry of illness-induced hyperalgesia, Brain Research 639 , 283–299.
Download references
Acknowledgment
We thank Dr. Lyn Lowry for her editorial support. The authors’ work is supported by NIH Grants AT002605, NS060735, and DE11964.
Author information
Authors and affiliations.
Center For Integrative Medicine, School of Medicine, University of Maryland, Baltimore, MD, USA
Rui-Xin Zhang
Department of Neural and Pain Sciences, Dental School and Program in Neuroscience, University of Maryland, Baltimore, MD, USA
You can also search for this author in PubMed Google Scholar
Corresponding author
Correspondence to Ke Ren .
Editor information
Editors and affiliations.
, Department of Anesthesiology, Yale University School of Medicine, Cedar Street 333, New Haven, 06520-8051, Connecticut, USA
College of Medicine, Department of Anesthesiology, University of Cincinnati, Albert Sabin Way 231, Cincinnati, 45267-0531, Ohio, USA
Jun-Ming Zhang
Rights and permissions
Reprints and permissions
Copyright information
© 2011 Springer Science+Business Media, LLC
About this protocol
Zhang, RX., Ren, K. (2011). Animal Models of Inflammatory Pain. In: Ma, C., Zhang, JM. (eds) Animal Models of Pain. Neuromethods, vol 49. Humana Press, Totowa, NJ. https://doi.org/10.1007/978-1-60761-880-5_2
Download citation
DOI : https://doi.org/10.1007/978-1-60761-880-5_2
Published : 07 September 2010
Publisher Name : Humana Press, Totowa, NJ
Print ISBN : 978-1-60761-879-9
Online ISBN : 978-1-60761-880-5
eBook Packages : Springer Protocols
- Publish with us
Policies and ethics
- Find a journal
- Track your research
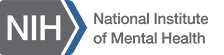
Transforming the understanding and treatment of mental illnesses.
Información en español
Celebrating 75 Years! Learn More >>
- Science News
- Meetings and Events
- Social Media
- Press Resources
- Email Updates
- Innovation Speaker Series
Day One: Placebo Workshop: Translational Research Domains and Key Questions
July 11, 2024
Welcome Remarks
ERIN KING: All right. We'll go ahead and get started. On behalf of the co-chairs and the NIMH planning committee, I'd like to welcome you to the NIMH Placebo Workshop: Translational Research Domains and Key Questions.
Before we begin, I'm going to quickly go through a few housekeeping items. All attendees have been entered into the workshop in listen-only mode with cameras disabled. You can submit your questions via the Q&A box at any time during the presentation. And be sure to address your question to the speaker that you'd like to respond. For more information on today's speakers, their biographies can be found on the event registration website.
If you have technical difficulties hearing or viewing the workshop, please note these in the Q&A box and our technicians will work to fix the problem. You can also send an email to [email protected]. And we'll put that email address in the chat box. This workshop will be recorded and posted to the NIMH event website for later viewing.
Now I'd like to turn it over to the acting NIMH Director, Dr. Shelli Avenevoli for opening remarks.
I think the audio is still out. If we can restart the video with the audio turned up.
TOR WAGER: That was some placebo audio. I think I might be able to share my screen and get the audio to come up on the video. So maybe I can try that. Hopefully you can see this okay. Let's see if it comes through.
SHELLI AVENEVOLI: Good morning. I'm excited to be here today to kick off the NIMH Placebo Workshop. I am currently the Acting Director of NIMH, and I look forward to serving in this role while NIMH conducts a national search for the next NIMH Director.
Today we are bringing together experts in neurobiology, clinical trials and regulatory science to examine placebo effects in drug devices and psychosocial interventions. NIMH has long understood that the placebo phenomenon is highly active in studies of mental illness. Understanding how to design and interpret clinical trial results as well as placebo neurobiological mechanisms have been important research questions that still have significant gaps. Subsequently, I'm eager to learn what you believe are the most important questions of placebo research and how they might be answered. This is no small charge, I understand. But our organizers have designed a carefully thought out agenda to help facilitate our success.
The workshop is organized into domains that aim to identify those important questions. I'm looking forward to hearing a historical review of the successes and failures around mitigating the placebo response in both academic and industry research. This includes historical perspectives in drug and device trials, understanding psychosocial aspects of the placebo response and measuring and mitigating the placebo effect.
Clearly, several perspectives will be discussed during these presentations. It will be exciting to hear your individual views as well as the panel discussions. I'd like to thank Doctors Tor Wager and Cristina Cusin, the co-chairs of the workshop, as well as the rest of the planning committee for their work in organizing this excellent agenda.
I will now turn it over to Dr. Tor Wager. Thank you.
Introduction and Workshop Overview
TOR WAGER: Okay. Hi, everybody. Sorry the audio didn't turn out as well as we had hoped, but I hope you could still hear it to some degree. And I just want to say I'm really delighted to have you all here. And I'm really delighted that NIMH has decided to organize this workshop and has worked so hard in planning it.
I'd like to thank my co-chair Cristina and also the NIHM co-leads Erin King and Doug Meinecke as well as the rest of the team that's been working really hard on preparing this meeting, including Meg Grabb and Laura Rowland and Alex Talkovsky, Mi Hillefors and Arina Knowlton.
My job for the next few minutes is just to give you a brief overview of the -- some of the main concepts in the placebo field altogether. And I'm going to start really at the very, very beginning.
The workshop goals are really to understand how placebo and nocebo effects impact clinical trial design and outcomes; to understand some of the psychological, neurobiological, and social mechanisms that underlie placebo effects.
And we'd like to think together to use this understanding to help to identify and maximize therapeutic effects of drugs and devices. And that means better clinical trial designs, better identification of outcomes, and also to harness placebo mechanisms in clinical care alongside active treatments so that we don't think of only specific treatments, we think of treatments as having psychological and psychosocial components as well as active drug or device components.
And to go back to the very, very beginning, my colleague Ted Kaptchuk once wrote that the history of medicine is the history of the placebo effect. So this is the Ebers Papyrus circa 1500BCE and it documents hundreds of ancient medications that are now thought to be little better than or no better than placebo effects. Some of them we recognize today like, for example, opium, the ingredient of opiates; and wormwood, the ingredient of absinthe for headache.
If you were poisoned, you might be treated with crushed up emerald or Bezoar stone which is undigested material from the intestines of animals. You might be treated with human sweat and tapeworms and feces, moths scraped from the skull of a hung criminal, or powdered Egyptian mummy, among many other treatments. And what all of these have in common is that none of them or very few of them have active ingredients in terms of specific effects, but they all act on the mind and brain of the perceiver. And so there is something about the beliefs and the imagination of the person that has made these treatments persist for many, many centuries.
And this provides both a challenge and an opportunity. I'm going to introduce the challenge with this clinical trial which is a gene therapy for Parkinson's disease AZ neurokinin which was an industry funded trial. And they went out two years. And this is a genetic manipulation intervention for Parkinson's disease. And what you see here is an improvement in motor scores in PDRS3 on Parkinson's. And if you see, people getting the active treatment, they got substantially better within the first six months and they stayed better for two years.
And this seems great. But the problem is that this trial failed. And the failure resulted in the drug company being sold off and this treatment may never see the light of day. And that's because people in the placebo group also got better and stayed better for two years. And there was no drug placebo difference.
And this is really shocking to me because Parkinson's is a neurodegenerative disorder. And so it's very surprising to see changes of this magnitude last this long. So the opportunity is in harnessing these psychosocial processes and the active ingredients that go into the placebo index like this, or placebo responses like this I should say. And the challenge, of course, is that placebo responses can mask effects of treatment in the way that we've seen here.
And this is not a unique occurrence. In many cases, there are treatments that are widely used that are Medicare reimbursed that turn out after they are tested later to not be better than placebo in clinical trials, randomized trials. And this includes arthroscopic knee surgery for arthritis, vertebroplasty, epidural steroid injections which are still practiced widely every day. Some other interesting ones like stents for angina, which is chest pain. And also some recent high profile failures to beat placebo after very initially promising results in emerging treatments like gene therapy for Parkinson's disease that I mentioned before and deep brain stimulation for depression.
A recent interesting case is the reversal of FDA approval for phenylephrine which is a very common nasal decongestant. It's the most widely used decongestant on the market. Almost $2 billion in sales. So it turns out, it may not be better than the placebo. One of the problems is that in some areas like, for example, in chronic pain, placebo effects are growing across time but drug effects are not. And so the drug placebo gap is shrinking and fewer and fewer treatments are then getting to market and getting through clinical trials.
And that's particularly true in this study by Alex Tavalo in the United States. So as an example, surgery has been widely practiced first in an open label way where people know what they are getting. And it was only much later that people started to go back and do trials where they would get a sham surgery that was blinded or just a superficial incision then. So the person doesn't know that they are not getting the real surgery. And those sham surgeries in many cases have effects that are substantial and in some cases as large or nearly as large as the active placebo -- as the active drug effects.
So this is what we call placebo response which is overall improvement on placebo. It doesn't mean that the sham surgery or other placebo treatment caused them to get better.
And so if we think about what the placebo response is, it's a mixture of interesting and uninteresting effects including regression to the mean, people fluctuate in their symptoms over time. And they tend to enroll sometimes when the symptoms are high. And there is sampling bias and selective attrition. There is natural history effects. And then there is the placebo effect which we'll define as a causal effect of the placebo context.
And the simplest way to identify a placebo effect is to compare placebo treatment with a natural history or no treatment group in a randomized trial. So here in this three-arm trial, a parallel groups trial, what you see is the typical way of identifying the effect is the active drug effect comparing active treatment to placebo. And you need to compare placebo to the natural history group to identify the placebo effect here.
And if we look at those studies that do such comparisons, we can see that there are many effects across different areas. And those effects are active brain body responses or mental responses to the treatment in context. And so there are many ingredients. It's not the placebo drug or stimulation or device itself, of course, that has the effect. It's the suggestions and the context surrounding that.
And there are many types of cues. There are verbal suggestions, information, there are place cues, there are social cues including body language and touch. There are specific treatment cues that are associated with the drugs. And there is a rich internal context. Expectations about treatment outcomes, interpretations of the meaning of what symptoms mean and the meaning of the therapeutic context and the care context. As well as engagement of emotions and memories. And what I'm calling here precognitive associations that are learned or conditioned responses in the brain and the body. So there is a large family of placebo effects; not one, but many placebo effects. They operate both via conscious and unconscious means. They are embedded in the nervous system through learning processes. And an idea here is that meaning of the response to the treatment to the person and the symptom is really the key. What are the implications of the cues and the symptoms and the whole context for future well being? o if we look at studies that have isolated placebo effects compared to no treatment, we see that there are many studies and many systematic reviews and meta analysis including many types of clinical pain in depression, in Parkinson's disease, in motor symptoms as well as other symptoms. In anxiety including social anxiety in particular and general anxiety. Substance misuse and perceived drug effects. Some effects in schizophrenia. Potentially some effects in asthma. And that is a sort of a tricky thing with the conflicting results that we could talk about. And effects on sleep and cognitive function and more. So these effects are really widespread.
There have been some attempts to decompose these into, you know, how large are the effects of placebo versus the effects of active drugs. And so if you look at pharmacotherapy for depression, at least in one analysis here by Irving Kirsch, half of the overall benefit, the placebo response -- or the active treatment response, I should say, is placebo. A very small proportion is specific drug effects. And about a quarter of it is people who would have gotten better anyway, they recover spontaneously from depression. That's natural history.
So the placebo effect is a large part of the overall therapy response. And this mirrors what's called common factors in psychotherapy. And common -- and this is for mood and anxiety disorders, substance use disorders and more. And common factors are those therapeutic elements that are shared across many treatments. And really in particular to -- they include drug and therapy, providing listening and social support, positive engagement and positive expectations. And in this analysis here the common factors also were responsible for a lion's share of the therapeutic effects of psychotherapy.
So in one sense you can say that placebo effects are really powerful, they can affect many kinds of outcomes. But there is continuing controversy, I would say. Even though these competing "New York Times" headlines are somewhat old now. And this latter headline came out after a landmark meta analysis in Froberg, Jenning, Kaptchuk in 2001 which they've updated several times since then.
And what they found is consistent with what I said. There are significant placebo effects in the domains that they were powered to detect. But they discounted those. They said it's probably due to reporting bias and other kinds of biases. So this is a key question is which outcomes count as important?
So here is an example from a fairly recent study of expectancy effects in anxiety. They compare it, people getting an SSRI in a typical open label way which is in the blue line with people who got a hidden SSRI, they didn't know that they were getting the SSRI. And that difference is a placebo-like effect or an expectancy effect.
There was a substantial drop in anxiety that was caused by getting the knowledge that you -- that people were being treated. So the question is does that actually count as a meaningful effect? And, you know, I think there's -- it's right to debate and discuss this. It relates to this idea of what I'll call heuristically depth. That this effect might simply be people telling us what we want to hear. That's a communication bias or a so-called demand characteristic that's been studied since the '50s.
It could be an effect on how people feel and their decision making about how they report feelings. It could be an effect on the construction and anxiety in the brain. It could be an effect on -- a deeper effect in potentially on some kind of lower level pathophysiology, some kind of effect on the organic causes of anxiety.
So the gold standard has been to look for these organic causes. And it gets very tricky when you define outcomes in terms of symptoms. Like is true with pain, with depression-related symptoms, anxiety-related symptoms and more in mental health. In pain, what the field has been trying to do is to look at pathways that are involved in early perceptual effects of nociception and on those central circuits that are involved in constructing the pain experience to ask if those are affected. And what we've seen, this is sort of the most developed area I think in human neuroscience of placebo effects. And we see reduced responses to painful events in many relevant areas. Including in the spinal cord areas in some studies that are known to give rise to nociceptive input to the brain.
There is increases in activity in punitive pain control systems that send descending projections down to the spinal cord. And there is release of endogenous opioids with placebo treatment in some of those pain control systems and other areas of the frontal cortex and forebrain. So these are all causal effects of placebo treatment that seem to be relevant for the construction of pain.
And what is remarkable is that the effects in the frontal cortex that are the most reliably influenced by placebo including the medial prefrontal cortex and the insula and other areas really are not just involved in pain, of course. They really affect some systems that are involved in high-level predictive control of motivation, decision making and perception.
So an emerging concept is this idea that what these circuits are for and what a lot of our brain is for in general is forming a predictive model of what is going to happen to us, what situation do we find ourselves in. So these cortical circuits are important for representing hidden states that we have to infer. And that's another way of saying meaning. Therefore, understanding what the meaning of events is. If it's an eye gaze, what is the meaning of that look? If it's a movement, what is the underlying meaning of the movement?
And it's that underlying situation model, predictive model that guides how we respond to a situation and what we learn from experience. So these systems in the brain that are influenced by placebo provide joint control over perception, over behavior and decision making including whether we choose to smoke or not smoke or eat more or eat less. And the body through the autonomic and neuroendocrine and immune systems. So broadly speaking, there is this joint control.
So this is one example where we can get closer to pathophysiology with some forms of placebo effects. And this is forebrain control over all of the various brainstem and spinal centers that are important for particular kinds of regulation of the body. The respiratory muscles, the heart, the intestines, and immune responses as well. When we look in the brain, the most consistent correlates in meta analyses of immune changes in the body are those that seem to play central roles in placebo effects as well like the ventromedial prefrontal cortex.
And another important development in this and aspect of this is the idea of parallel models in nonhuman animals and in humans, particularly those that use classical conditioning. So there are many kinds of pharmacological conditioning in which a cue is paired with a drug over time, usually over several days. And then the cues alone like the inscription alone can come to enlisted effects that sometimes mimic drug effects and sometimes are compensatory responses that oppose them.
And one of the most famous was the phenomenon of conditioned immunosuppression that was first published by Bob Ader in 1976 in Science and has since been developed quite a lot. So this is from a review by Mia Chelowoski's group which is a very comprehensive review of different kinds of immunosuppressive responses. And the point I want to make here is that there is increasing evidence that the insular cortex as an example is really important for storing memories about context that then get translated into effects on cellular immunity that are relevant for the trajectory of health and disease in broad ways. And those areas of the insula are similar to those that are involved in placebo effects in humans on pain, itch, cough, disgust and other conditions as well. So there is the potential here for memories that are stored in the cortex to play out in very important ways in the body. And that can influence mental health directly and indirectly as well.
And I want us to move toward wrapping up here with a couple of ideas about why these effects should exist. Why do we have placebo effects in the first place? And two ideas are that we need them for two reasons. One is for predictive control. The idea about what we need an evolved brain for, a highly developed brain is to anticipate those threats and opportunities in the environment and respond in advance. So it's not that we -- we don't respond to the world as it is. We really respond to the world as it could be or as we think it will be.
And the second principle is causal inference. That we -- what is less relevant is, is the particular sensory, you know, signals that are hitting our apparatus at any one time. And what is really more important is the underlying state of the body and the world, what is happening.
Just to illustrate those things, one example from Peter Sterling is this very complicated machinery for regulating blood pressure when you stand up and when you are under psychological stress. And we need this complex set of machinery in order to predict what the current -- what the future metabolic demands are. So our blood pressure essentially like other systems responds in advance of challenges. And that's why we get stressed about a lot of physiology.
An example of the second is a simple example from vision. If you look at these two squares that we circled here, you can see they probably look like they are different colors. One is brighter and one is darker. But if I just take away the context, you can see that the squares are exactly the same color. And so you don't see the color of the light hitting your retina. What you see is your brain's guess about the underlying color of the paint or the color of the cubes that discounts illumination and factors it out as a cause. So what our perceptual systems are doing is causal inference.
So with pain, itch or nausea, for example, other symptoms, you don't -- or mood or motivation, you don't feel your skin or your stomach or your body in a direct way. Your brain is making a guess about the underlying state from multiple types of information. And this really starts with our memories and past associations and our projections about the future.
So I'm using pain as an example because we study it a lot. But the idea is that the pain really starts with these projections about the future. And there is a representation in the brain of the current state of threat and safety, if you will. Nociceptive input from the body plays some role in that, but it's really the central construction that integrates other forms of context, what is the look, what kind of support are you getting, that together determines what we end up feeling.
And there are different kind of responses that are linked to different parts of that system. But the idea of suffering and well being, of fatigue and motivation, all of those things I think are related to the current state.
There are many open questions. You know, one is which outcomes count as important for determining whether an intervention is meaningful? Can we separate changes on decision making and suffering from response biases that we really shouldn't consider important for clinical research.
Secondly, can we identify outcomes affected by real treatments, drugs and devices but not placebos? And how can we use those outcomes in clinical trials in advance of the regulatory front as well on the scientific front?
Third, what kinds of experimental designs will help us separate specific effects from these broader context effects? And is this a reasonable goal? Can we actually separate them or do they often work together or synergize with one other? So do they interact?
Fourth, can we predict who will be a placebo responder from personality, genetics perhaps, or brain responses? Can we use this to maximize our treatment effects in clinical trials and improve the pipeline? And, you know, unclear whether that is possible.
And finally, how can we use all of these factors we've discussed alongside other treatments that are current medical treatments to improve outcomes?
With that, I'm just going to introduce the next -- the rest of today. I realize we're a little bit long getting started. Hopefully we can make up some time here. But now we're going to start our first session which is about perspectives on placebo in drug trials from Michael Detke and Ni Khin and Tiffany Francione. So this is going to be about the sort of history and state of how placebo effects interface with the regulatory environment.
Then we'll take a break. And after that we'll continue to the rest of the sessions. So without further ado, I would like to turn it over to Mike. Thank you.
Historic Perspectives on Placebo in Drug Trials
MICHAEL DETKE: I think Ni is going before me. Correct, Ni?
NI AYE KHIN: Yes, I am.
MICHAEL DETKE: Okay, thank you.
NI AYE KHIN: I'll do the first part for the historical perspective.
Hi, I'm Ni Khin. And I'll be talking about historical perspective on placebo response in drug trials.
My disclaimer slide. Although I'm currently an employee of Neurocrine Biosciences, part of the presentation today is the work conducted during my tenure with U.S. Food and Drug Administration.
The presentation reflects view of my view and it's not being not quoted with all the organizations that I was affiliated with and currently affiliated.
Let me start with a brief overview of what FDA required for drug approval. FDA regulation defines that there should be substantial evidence, evidence consisting of coming from adequate and well-controlled trial.
The usual interpretation is that it would require two positive randomized controlled clinical trials. However, in terms of drug approval process, we use holistic approach in review of clinical efficacy and safety coming from clinical trials. So in FDA data from both successful and non-successful study, positive and negative studies, as a package when the industry or the drug sponsors submit New Drug Application packages to the agency. And these mainly the efficacy results generally would come from shorter term efficacy data. And safety data will be according to the ICH requirement 1500 patients, three to 600 for six months and at least 100 patients for a year. Generally the maintenance efficacy or also relaxed prevention trials are conducted mostly post approval in the U.S.
So the data that I'm presenting was conducted as kind of a pool analysis from the data that was submitted to agency in terms of in support of New Drug Applications. Why we did that data mining effort. And as you know high rate of placebo response and decline in treatment effect is over time in psychiatry was the main major concern. At the time when we did this analysis if there were increasing trials at clinical trial sites outside the U.S. And we are looking into applicability of such data from non-U.S. sites in the U.S. population.
So we did exploratory analysis of pooled efficacy data from two different psychiatric indication, major depressive disorder and schizophrenia. We have data level coming from trial level and subject level data. And we for depression across the application package, we have Hamilton Depression Rating Scale as the common primary or key secondary efficacy rating scale. And schizophrenia application packages we have PANSS which is Positive and Negative Syndrome Scales.
So we were looking at those two endpoint measures. And then did some exploratory analysis and then summary from these findings. And the processes and challenges experienced in our effort looking into these databases will be shared today.
Let me start with depression trial level data that we looked at. It consisted of 81 RCT short-term trials. So it spans about 25 years. So these are mainly SSRIs and SNRIs, antidepressant. From that 81 short-term control trial, total number of subject was over 20,000 subject, 81% enrolled in U.S. sites. And as you could see here, majority were whites, Caucasian, female. And mean age was around 43 years of age. And baseline HAMD scores were approximately 24. And dropout rate, average dropout rate in these trials was approximately 33%.
We explored treatment effect and trial success rate based on the questions raised about applicability of data from non-U.S. site to the U.S. population. This is the overall results that we published in 2011 paper. We noticed that both placebo and drug group from non-U.S. tended to be larger change from baseline in HAMD-17 total scores than those observed in the U.S.
You can see on the left-hand column non-U.S. site placebo response is approximately 9.5 and U.S. is 8. But drug effect were also larger slightly in non-U.S. sites and U.S. is slightly lower. So if you subtract drug placebo differences, average is about the same for both U.S. -- data coming from both U.S. and non-U.S. sites. So it's about 2.5 points HAMD total difference.
So what we see overall over 25 years of antidepressant trials is that there is increase in highly variable placebo responses across trial. Slight decline in treatment effect moving from approximately three points difference in HAMD total towards two points drug and placebo difference. In trial success rate was slightly lower, 55 versus 50.
And as part of that analysis we also look at any difference in data between fixed and flexible doses. So 95% of the trials that is in the database utilize flexible dosing design regimen. And so placebo responses were quite similar. Treatment effect was slightly larger for flexible doses as compared to fixed dose.
And we pointed out that in our analysis we used data versus -- data coming from the treatment arms versus number of trials as the denominator in the calculation. So slightly higher trial success rate for fixed dose trials, which is 57%, versus flexible dose 50%.
So and some of you may already know that there was an earlier paper published by Arif Khan and his group. A similar database, but it was datasets coming from trial conducted between 1985 to 2000.
And from that analysis it was showing that 61% of the flexible dose studies versus 33 for fixed dose results in terms of success rate. And Khan's use number of treatment arm as the denominator. And if you look at the results, it's a flexible dose is also 60% compared to 31% of fixed dose. However, in our larger database, data included conducted after 2000, that is 2001 to 2008, our findings are in favor of still fixed dose design with success rate around 60% for fixed dose arm, compared to 34% for flexible dose arm. So we think that the more recent trial fixed dose studies, the success rate is likely higher.
So in addition to trial level data, we also look into subject level data from these trials. So for subject level data we initiated with 24 randomized control trial data from -- then we expanded to 45. And the main thing that we were looking at was the – what could we use in terms of responder definition. Do we need a HAMD total cutoff?
So from that analysis we noticed that overall 50% change for baseline is sufficient to define responder status and HAMD total cutoff is not necessary. Whether you use percent change or HAMD total cutoff or both, we would capture more or less the same folks as the responder, median responder status.
And then another item that we looked into was for optimal trial duration. And we -- if you -- from -- generally from eight weeks trials are the ones that would give overall successful trial results. And we looked into whether if we shorten it to six weeks, whether it will get similar results. So it was like somewhere in between that maybe shorten if you could see the two points difference at week six.
And another item that we look into was time to treatment discontinuation instead of change from baseline as the primary efficacy endpoint. And the data support -- not supportive of time to treatment discontinuation as an alternative primary endpoint for drug trials.
So I'm going to cover a little bit about efficacy results from maintenance efficacy trials also known as relapse prevention trials where we usually use randomized withdrawal design.
And they are generally not regulatory requirement in the U.S. to do maintenance efficacy study. But if the agency would see it would be needed, then we'll communicate with the drug sponsor before coming in with the application.
So as you could see on this slide, these longer term maintenance efficacy study generally design as open label treatment for approximately 12 weeks. Once they meet the stable responder status will be randomized into double-blind randomized withdrawal phase to either continue on the drug or the other half will be into placebo. The endpoint generally used is the time to relapse or relapse rate. And we did overall look at trial level data from 15 randomized controlled maintenance, randomized withdrawal trial that was conducted between 1987 and 2012. And you can see demographic disposition is more or less the same for this trial. Average number of subject per study is in the 500. And mean HAMD score at baseline prior to open label is more or less the same. And randomization after they meet responder status to drug and placebo HAMD total score is 9.4.
And the relapse and -- response and relapse criteria used in these studies are varied among studies. And stabilization period is varied. Regardless of that, these are approved based on short-term study. You also see maintenance efficacy based on the results of this study.
This is just the overall slide that shows the duration of open label -- open label response criteria, response rate, double-blind study period, relapse criteria, and different placebo relapse rate and relapse rate and 50% reduction in terms of relapse difference you will see with the drug treatment.
These results were published. And overall I just want to summarize the results saying that almost all the trials are successful. Open label phase, mean treatment response is about 52%. Those meeting responder status going into double-blind randomized withdrawal phase, there is average 50% reduction in relapse rate for drug treatment group as compared to placebo. And in that paper we have side by side comparison of subject level data in terms of relapse survival analysis Kaplan-Meier Curve.
And let me summarize a little bit about schizophrenia trial data. We did have a pool analysis of 32 randomized placebo-controlled short-term clinical trial that was conducted between '91 and 2009. And those are mainly atypical antipsychotics. And this slide shows number of subjects along with mean age and demographic distribution along with the mean baseline PANSS total score.
And we provided the observed increasing placebo response, stable drug response, and declining treatment effect over time in North America region. One thing we would notice was that treatment effect decrease as body weight increased in North America trial patients. And this is FDA also conducted post 2009 period analysis. And this slide shows comparison between pre 2009 trials and post 2009. And you could see that predominantly multiregional clinical trial in recent years dropout rate is higher, slightly higher. But continuing trend of increasing placebo and decreasing treatment effect when you look at in combination of two different pool analysis is that it still persist over 24-year period. Those both level pool data analysis and schizophrenia data analysis is for 25 years period.
So I'm just going to let folks know a little bit about challenges in doing these type of pool analysis is the datasets. Data standard issue. And it was because of the technology in those times' difference. We do not have subject level data trial conducted before 1997 in the database.
And of course always the resources is an issue. And the main point that I would like to bring for everyone's attention is the collaboration, collaboration, collaboration in terms of solving this major issue of placebo response.
I'm going to stop here. And I'll let Dr. Mike Detke continue with this topic from industry perspective. Mike.
MICHAEL DETKE: Thanks, Ni. I'm having problems sharing my screen. I got to make this full screen first. Okay, great. Sorry, minor technical problems. Thanks for the introductions, thanks to NIMH for inviting me to present here.
As Ni said very well, my background is industry. I'll be presenting this from kind of an industry perspective. I've spent 25 years working at a clinical trial site at big pharma, small biotech, and a vendor company all in CNS clinical development, mostly drugs. And I'll -- I'm also a board certified psychiatrist and practiced for about 20 years. I still do medicine part time. And I'll talk about relevant disclosures as they come up during my talk because I have worked in these fields a fair bit.
So that being said, there we go. This is just a high level overview of what I'll talk about. And again, from the industry perspective in contrast to the –
ERIN KING: Your camera is off if you want to turn it on.
MICHAEL DETKE: I will turn it on. My apologies. There we go.
So as I said, I'll be presenting from the industry perspective. And for the most part my definition of placebo response throughout this talk is if the patient got seven points better on placebo and the patients got ten points better on drug, the placebo response is seven points and we'll be focusing on that perspective.
And Tor gave a great overview of many other aspects of understanding placebo. And we'll talk and my esteemed co-presenters will talk more about that, too.
But again, I'll give you the historical perspective. And mostly I'm going to try to go through some data. Some a little older, some a little newer, that of things that have been tried to reduce placebo response and/or improve signal detection, drug placebo separation which especially in a proven effective therapeutic is probably a better way to look at it. And this is just a list of some of the topics I'll cover. I've got a lot of ground to cover, and this won't be exhaustive. But I'll do my best to get through as much of it as possible for you today.
Dr. Khin already talked about designs including the randomized withdrawal design. Important to keep those in mind. I'll briefly mention a couple of other major designs here that are worth keeping in mind.
The crossover design has an advantage that it's much higher statistical power because in -- the ideal way to use this is to use the patients themselves as their own control groups. So you're doing within the subject statistics which make this much more powerful. You do a much more statistically powerful study with far fewer patients.
A couple of important cons are there can be washout effects in the drugs. So pharmacokinetic or even if it's completely washed out, the patient's depression or whatever might have gotten to a better state that might be lingering for some time. And because of these overlap effects there, you can't be totally certain that the baseline of phase two is the same as the baseline of phase one. And that's an important issue. And those overlap effects are important.
But diseases with stable baselines and I think in the CNS space things like adult ADHD could be things that you would consider for this perhaps in proof of concept rather than confirmatory, though. I'll leave that to my colleagues from the FDA.
Sequential parallel design. This has been presented a long time ago and published on much. This is a design where some of the patients get drug in the phase one and others get placebo. They randomize just like a typical parallel arm randomized study. However, in a second phase the placebo nonresponders specifically are then re-randomized to receive placebo or drug. So this has a couple of advantages.
One is that there are two phases from which you can combine the data. And the other is that this second phase enriches for placebo non-responders just like the randomized withdrawal enriches for drug responders. And this has been published on in the literature. This is a slide that hasn't been updated in a while. But the results even back a few years ago were, you know, out of, you know, quite a few trials that have been reported on.
There was a reduction in placebo response in phase two. The drug placebo difference improved. And the p values were better and so forth. So this is an important trial design to know about. Dr. Farchione will talk about I think one example of this having been used recently. It's a little bit hard because you can't really do this within trial comparisons of different trial designs. That's a limitation.
So these are all cross-trial comparisons really. But and there are some advantages and disadvantages. It -- by using patients twice, you might be able to do the trial with somewhat fewer patients, save money, save time. On the other hand, there is two phases so in that sense it might take a little longer. So various pros and cons like anything.
And then I'm going to talk about placebo lead-in. So historically people did single-blind placebo lead-ins where all patients would get placebo for the first week or so blinded to the patient, not to the staff. And then if they had a high placebo response, they would be excluded from the study.
Typically it was about a week and about a 30% placebo response, but it varied. Trivedi & Rush did a great review of this, over a hundred trials as you can see. And little evidence that it really improved -- reduced placebo or improved drug placebo separation. This is some work from my early days earlier in the 2000s at Eli Lilly when I worked on Cymbalta and Duloxetine for about seven years. We did something called a variable duration placebo lead-in where we -- this was the design as it was presented to the patients and to the site personnel that randomization would occur anytime between week -- visits two and four. Which meant they were on placebo for either 0 to one to two weeks. Usually, in fact, they were on for one week.
This has some pros and cons again practically. This -- the placebo lead-in adds a week or two of timeline and cost. The patients, the way this was designed and to maintain the blind, the patients that you, air quotes, throw out for having too high of a placebo response have to be maintained throughout the study which costs money and means that your overall end might need to be higher. So time and money implications.
When we looked at this, Craig Nalstrom, a statistician published from this. And we found that the average effect size did go up pretty substantially, this is going to the effect size. But you also lost some end when you excluded placebo responders. So the frequency of significant differences did not go up substantially in this analysis.
Moving on. Dr. Khin referred to this study by Arif Khan where flexible dose trials did better than fixed dose. I would say that, you know, the database that Dr. Khin presented from the FDA, bigger database, you know, less publication bias and things like that. So I would lean in favor of preferring that. But I would also say that if you focus on my last bullet point, there is clinical intuition about this. And ask yourself the question if you had a case of depression and you could go see a doctor that would only prescribe 20 milligrams of Prozac to every patient or a doctor that would prescribe 20 milligrams and if you're having side effects maybe titrate down, and if you're not having X he might titrate up, you know, which doctor would you rather go to?
So I think on some level it seems to have good faith validity that adjusting the dose to individual patients should lead to better efficacy and better assessment of true tolerability and safety. And that should do a better job than adjusting the dose of placebo. But importantly, because flex dose studies are two arms, one drug with a flexible dose and one placebo. And fixed dose studies are frequently dose-finding studies with, say, one arm of placebo and maybe three arms, 10, 20 and 40 milligrams of drug. So the number of treatment arms is practically, it's confounded with fixed versus flexible dosing. And likewise -- and that may matter. And the percentage randomized to placebo. And again, this is confounded with number of arms.
If you do equal randomization in a two-arm study, you have got a 50% chance of placebo; a four-arm study, you've got a 25% chance of placebo. And again, it makes good base validity, good sense that if your chance of getting placebo is much higher then you might have a higher placebo response rate or the chance of getting active drug is higher.
And that is what Papakostas found in a meta analysis in depression and Mallinckrodt again in a meta analysis of schizophrenia data. So those were all confounded. And they have pros and cons. And you do need to do some dose finding with your drug anyway. So they are all designs that have pros and cons to lead to better outcomes.
Better scales. This is a simple analysis taken from that same paper that did the double-blind placebo lead-in with Mallinckrodt. And we just looked at a pooled set of 22 RCTs. I think these were mostly or all duloxetine studies and depression studies. And the HAMD-17 item scale had an average effect size of about .38. But some of these subscales, which are, you know, five, six, seven or eight items long of items among the 17 in the HAMD. In other words, if you throw out half of the data from the HAMD, you could actually get a better effect size. And so this is something to think about at least in proof of concept. Obviously these subscales would need to be validated for regulatory and other purposes. But good to know that there are different approaches.
And too, if you have a drug that you believe based on earlier clinical data or preclinical data that are more likely to be efficacious in certain domains, symptom domains, that is important, too.
Statistical approaches. This is a little bit dated at this point in time, but there are a lot of important statistical issues to take into account. When I entered the industry, last observation carried forward, LOCF, was the gold standard. There have been a lot of papers published on mixed model repeated measure that protects better against both false positives and false negatives, gives you better effect sizes here. And here almost, you know, 30 or so percent bigger which is pretty substantial. And I'll show you that later. So better protection against false positives and false negatives means we have got more true positives and true negatives which is exactly what we want in therapeutic development.
And I'll talk here now about different implementation strategies during the trial. Central raters and a lot of people use different terminology here. So my terminology for central ratings is when a rater is remote and actually does the assessment. They are asking the questions, they're hearing the answers, they are asking for clarification, they are doing the scoring, etc. And these raters can be more easily blinded to protocol pressures and more easily independent of pressures to meet enrollment and so on and so forth. Note here, I was previously an employee and stockholder and consultant to MedAvante which was one of the companies that pioneered doing the central ratings. So I'm no longer -- I don't have any stock or no financial conflicts of interest now, but I did work with them for a while.
One advantage to centralized ratings on the right is that you can simply use fewer raters which reduces the variance that all of us humans are going to contribute. These people can be trained together more frequently and more consistently. And that can reduce variability, too.
Just some perspective, and Tor presented some nice stuff from other therapeutic areas, too. Is that, you know, in psychiatry, in CNS most of our outcomes are subjective and highly variable and probably need to be improved upon in some ways. Despite that, in other areas where there is probably less inherent variability, they have already standardized the fact that, you know, centralized blinded review or assessments by at least a second or a third person for lots of other types of therapeutics. And these are relatively old guidances from the UMA and FDA mandating this in other therapeutic areas.
So then to get back to the data on centralized ratings, MedAvante was able to conduct about seven studies where they did within study comparisons of site-based ratings and centralized ratings. And across these seven studies, my interpretation, and you can look at the data, are that about five of seven were green. They were clearly -- clearly showed better lower placebo responses or if there was an effective drug, better drug placebo separation with centralized ratings. And two showed pretty equivocal or not impressive differences.
And again, I'm a former employee and consultant to MedAvante. Here is one example, a large GAD study with -- that had escitalopram as an active comparator. And you can see the effect size was about twice as big in HAM-A points. The Cones-D effect size here was about twice. And the chart we put together when I was at MedAvante illustrates that a doubling of the Cone-D effect size means that you can either reduce your sample size by 75% and still have the same statistical power; or you can select a sample size of, say, N of 100 and your power goes up from about 60 to almost 100.
The more important way to read these powers is that your chance of a false negative, your chance of killing your drug when you shouldn't have is 38% with this effect size. And less than 1% with this effect size.
So then there are other approaches than having a central rater really do the assessment remotely. You can review the work, have a third party review the work of the site-based raters. MedAvante, their competitors Verisite, Signed and others all offer these services now and other companies do, too. And I'm not trying to -- and I don't know of any reasons to prefer one versus the other.
So you can review the source documents, audio or video recordings. This looks like it should work. It has good face validity. I've run trials with this. But I'm just not aware of any control data. I haven't seen studies where people have done third-party remote feedback in, say, half the sites or half the raters and not the other half and shown results. If you have those data, please send them to me. I'd love to incorporate those.
But, as I said, it has good face validity. You know, if you're giving people feedback on the quality of their assessment, the raters should do nothing but improve. There is effect called the Hawthorne effect that people behave differently when they know they are being monitored. This should work.
And let me talk a little bit about operations, doing central ratings is pretty burdensome. You have got to coordinate ratings with a rater that's somewhere else maybe in a different time zone and the patient and the site. It's expensive. It's labor intensive. This is less labor intensive because you don't have to review all the recordings. It can be done not in real time. And so it's less burdensome, it's less expensive.
Not clear exactly how efficacious it is, but it has good face validity. Or just replace those human raters with computers. There have been a lot of different groups that have done work on this. And I'm going to jump right into some data.
These are data from -- you'll recognize duloxetine again. And John Grice was one of the early pioneers in this in a company called Healthcare Technology Solutions. And this was done with patient self-report using IVR. So just basically an old fashioned keypad on a phone is good enough to do this. And the patients self-report this. And for those of you that don't know this, separating 30 and 60 milligrams of Duloxetine is really hard. We never really saw this with clinical rating scales.
But patients self-rating using a computer in days saw really nice signal detection and really rapid signal detection. And this is just another example of a different measure, PGI. And again, really impressive separation on these. Or humans are good and computers are good, why not combine the both. And Gary Sachs founded a company called Concordance many years ago. And it's been merged into other companies. And this is part of Signed now. And they showed that if you did a clinician rating and a patient self-rating by computer and compared them, you could learn a lot from the points that were not -- were discordant. And you could learn a lot about both severity ratings but also inclusion/exclusion criteria, diagnosis, things like that. So that's valuable.
Let's talk about professional patients quickly. This is just an anecdote. And I generally stay away from anecdotes, but I found this is really compelling. This subject returned to the site with their unused pills from their pill bottle. Unfortunately, he had a pill bottle from a different trial site, same sponsor and protocol. And this is probably a common problem. This is a phase three program in depression where they had up to 4% duplicate subjects at least in screening. It could be higher. We don't know how big the problem is. But we know it's a kind of a -- it's a tip of the iceberg issue. Because you can look -- you know, there probably aren't too many patients that are bold enough to try to enroll twice at different sites in the same study, but they might enroll sequentially. They might go through multiple screenings until they get in. They might be in different studies by different sponsors for the same or even different indications. Be in a bipolar study this week and a schizophrenia study next month, and a depression study the month after.
And these patients may or may not be compliant with medications and also protocol features. Anecdotal data on subject selection. There are lots of websites out there that will teach you how to be a bad patient in a clinical trial. And I just want to note, not that it's that a bad thing, I love ClinicalTrials.gov, I use it a lot, but any tool can be used for good or bad things, or almost any tool.
And the reason I mention this to you again, as you are posting your trials on ClinicalTrials.gov you want to be transparent enough to share what you need to share, but you might not want to help them too much with specific details of certain inclusion/exclusion criteria that are subjective and can be, for lack of a better word, faked.
The top three of these are all companies that do duplication check for duplicate patients that might be in your study and another study that they have in their database. I've worked with all of them. And worth noting, this is relatively minimally expensive. You just have to get a few demographics on each patient at screening. So also the site and patient burden are pretty minimal.
And AICure is really more of a medication adherence platform. But of course the really bad professional patients don't want to take the medications either. So there is some overlap between professional patients per se and medication adherence. Medication adherence. I'm going to go through the rest of this quickly in the interest of time. Difficult to know with certainty. Not as helpful if done after randomization certainly if you need intent to treat. But pK collection is important. One way to do it is just pK collection. That is a gold standard that tells you that the drug is in the patient's body. I'm going to skip this slide, too.
If half the patients don't take their medicine, you can imagine that the power is very bad. And I did consult with AiCure previously. That's an important disclosure, too. The reason I like AiCure, not so much because I consulted with them, there are many medication adherence platforms out there on the market. This is the only one where I've seen evidence that their platform is consistent with, correlates with, predicts pK values. So if I were you, that's an important question to ask. Then you also have to ask about all of the operational issues, too.
Biomarkers. I mean when we've got biomarkers, they're great. You know, if you've got a PET ligand and you can -- help you narrow down the dose and really demonstrate that you are engaging the target, that's fantastic. This is just an example of PET ligand. This is another biomarker. This is hot off the press, this was presented just a few weeks ago at ASCP. And the idea here is basically taking baseline demographics and putting them all into an AI model to see what predicts placebo response and drug placebo separation.
This is another company that I work with currently so there is that disclosure with as many grains of salt as you believe. We did a blinded analysis of baseline EEGs and identified three clusters in a placebo-controlled Zoloft study.
In the overall study, it just failed to separate. And we identified three distinct clusters, one of which has a huge Cone-C effect size and P value even in a little less than half the population. Another cluster that really weren't responders at all. And a cluster, the third cluster that is less than 20% of the population that had fantastic placebo responders and terrible drug responders.
So this needs more validation like all biomarkers. And I just want to leave this with the point that biomarkers are great as we continue to understand the biology and pathophysiology better. First we are going to have to validate these against the gold standards. And the current gold standards are variable and biased and imperfect. So to close on a relatively optimistic note, this is a red, green, yellow. Green is good. Yellow is questionable. Red is probably not that worth it. My own personal subjective assessment of -- but the takeaway is that a lot of these things can be helpful, especially when fit for purpose with the therapeutic that you are developing, the phase of development, and your strategic goals for that therapeutic.
So I'll end there. Thank you very much for your attention. Look forward to questions and so forth.
TOR WAGER: Great. Thank you, Mike. For time reasons, we're going to go on to our next speaker. But just to let everybody know, there's a Q&A and people are posting questions there. And our panelists can answer questions there in the Q&A panel as well as in the -- during the discussion phase. So keep the questions coming, thank you.
All right. Dr. Farchione, thank you.
Current State of Placebo in Regulatory Trials
TIFFANY FARCHIONE: Thank you. Let me just get this all cued up here. So thanks, everybody, and good afternoon.
As we've already said, my name is Tiffany Farchione, and I'm the Director of the Division of Psychiatry in the Center for Drug Evaluation and Research at the Food and Drug Administration. So because I'm Fed, I have no conflicts to disclose.
I'm going to be providing the regulatory perspective of placebo response in psychiatric trials. So, so far today you've heard a little bit of an historical perspective from Dr. Khin, who is actually my former team leader and peer reviewer. And she showed us that not only do we have a high rate of placebo response in psychiatry trials, but the extent of that problem has actually been increasing over time.
And then Dr. Detke just presented some of the strategies that have been proposed for dealing with this problem. And in some ways they are somewhat limited utility in some examples.
So I'm going to talk a little bit about the importance of placebos for regulatory decision making and give a few examples of placebo response mitigation strategies and registration studies. And then I'll go on and talk a bit about placebo response in other disease areas and end with some thoughts on what may ultimately help us to resolve this issue. All right. So I want to start first by expanding a bit on Dr. Khin's presentation and just quickly presenting some updated data. I saw that there was a question either in the chat or the Q&A about depression studies. And honestly, we don't have too much more from what she presented in depression. And also the things that we've approved more recently have different designs, different lengths of treatment and things like that so it makes it hard to combine them with the existing dataset.
But here we've got figures for schizophrenia and bipolar. And they look a little different from each other because I pulled them from a couple of different presentations. But essentially the data points in each figure represent the change from baseline to endpoint on either the PANNS on the left, or the YMRS on the right in critical trials of atypical antipsychotic medications for the treatment of either schizophrenia or bipolar one disorder.
And the drugs included in these figures are ones for which we have both adult and pediatric data. So on the left you can see that the trend for increasing placebo response over time is also evident in the adolescent trials. And then on the right, we have data from adult and adolescent bipolar one studies, which Dr. Khin didn't present. So there are a few data points in this side, fewer than in schizophrenia. But the trend is less obvious from the dots alone. But if you draw in the trend lines, which are here on the figure, that allows you to see that the same phenomenon is also at play in the bipolar studies.
All right. So let's go back to basics for a minute and talk about why we need placebos in clinical trials in the first place. So simply put, placebo-controlled studies are our bread and butter. And in order to support a marketing claim, companies need to provide substantial evidence of effectiveness for their drugs. Ni went over this a little bit as well. This is generally achieved with two positive adequate and well-controlled clinical studies. And the characteristics of adequate and well-controlled studies are outlined in the Code of Federal Regulations.
So there's seven different characteristics that are listed in CFR, but one of those states that the study has to use a design that permits a valid comparison with a control to provide a quantitative assessment of the drug effect. So more often than not, that's a placebo control.
And they've agreed we just need some way to determine that the drug itself is actually doing something. So if the treatment response in the drug arm is greater than the response in the placebo arm, then that difference is assumed to be evidence of a drug effect. But that may be oversimplifying things just a little bit. It's important to remember that the difference -- the difference between an effect and a response. So the response is the observed result like the change from baseline on a PANSS or a MADRS score. And the drug effect can be one component of that. But adherence to the drug, timing of the assessment, other factors also influence the observed response.
And yes, a portion of the drug response is probably attributable to placebo effect. Same thing with placebo response. Yes, the placebo effect itself is a component of the response observed. But you also have things like the natural history of the disease or regression to the mean or, you know, when we talk about adjunctive treatment, it could be that the other treatment is part of that effect. All of those play a role in the observed response in a study.
So what exactly is it that can account for the placebo response rate in our client's trials? So Dr. Detke went over several of these examples earlier. But let's start with expectancy. And this is a big one. If folks expect to have some benefit from a drug that they're taking, they oftentimes do experience some benefit. The structure of a clinical trial can also contribute to the placebo response. Folks are being seen on a regular basis; they have a caring clinician that they interact with routinely. Those things can in and of themselves be somewhat therapeutic.
The fact that we use subjective outcome assessment is another aspect of this that I want to highlight. Because in psychiatry trials, we can't draw labs or order a scan to ensure that we have the right patients in our trials or to objectively assess their response to the drug. What we have are clinician interviews and patient reported outcomes. And oftentimes these outcome assessments involve a report from a patient that is then being filtered through a clinician's interpretation and then translated into a score on a scale. So there is a lot of room for variability in that.
The distal nature of that assessment from the actual biological underpinnings of the disease can be problematic and it's certainly prone to misinterpretation and to biases also. So again, Dr. Detke also mentioned how enrolling inappropriate participants can impact placebo response. If you have folks in a trial who don't actually belong in the trial, whether that's the professional patients that he kind of finished with, or whether it's folks who just don't quite meet the inclusion criteria or who have been misdiagnosed somewhere along the line, any number of things. That's going to increase the variability in your study and could potentially result in increasing the placebo response. So, of course, there's lots of other factors that can contribute to the placebo response. But because Dr. Detka spent a lot of time on this already, I just wanted to highlight these few skews.
So next I want to talk a little bit about ways in which we could potentially manage the placebo response in clinical trials. First, I want to present one option that we actually have not yet accepted for new drugs in psychiatry, but it's an option that actually takes placebo out of the equation entirely. We have a bunch of approved antidepressants, a bunch of approved antipsychotics. So at this point you might be asking why we can't just do non-inferiority studies and attempt to demonstrate that the new drug is no worse than some approved drug.
So the complicating factor here is that conducting a non-inferiority study requires defining a non-inferiority margin. And in a non-inferiority study, you are trying to show that the amount by which the test drug is inferior to the active control is less than that prespecified non-inferiority margin, which is M1.
And M1 is estimated based on the past performance of the active control. But, unfortunately, because of the secular increase of placebo response over time, we can't really estimate M1. It's a moving target. So even though we have things that have been approved in the past, we don't know that the margin by which the active drug was superior to placebo in the clinical trial that supported its approval is the same margin that would be observed today under similar circumstances. So because we can't set a non-inferiority margin, we can't do non-inferiority trials, at least not for regulatory purposes in psychiatry.
Another strategy that's been employed in a few trials at this point is sequential parallel comparison design. And again, Dr. Detke went over this briefly so you have some idea of the principles behind this already. Now recall that this is a design in which you have two stages. And the first is intended to weed out the placebo responders so that in the second stage the drug placebo difference is amplified --
So there is some statistical concerns with this type of study design related to the relative weights of the two stages and the impact of dropouts. But we have had one application where trials that employed the kind of trial design made it to the New Drug Application stage. And this application was presented at an advisory committee meeting back in November of 2018. So there is publicly available information for me to share even though the application ultimately was not approved.
This was for a fixed-dose combination of Buprenorphine and Samidorphan. So it was intended for the adjunctive treatment of major depressive disorder. Now the figure on the right-hand side was taken directly from the AC briefing book. And it shows diagrams of three studies in which SPCD was employed as part of the clinical trial setting.
The important thing to observe here is that you do in fact have a large placebo response in stage one and a much smaller placebo response in stage two. But what we don't see is the expected amplification of the drug placebo difference in stage two.
So as I said at the advisory committee meeting, either SPCD isn't working or the drug isn't working. So regardless of the outcome here, the important take home point is that we were able to file an application with SPCD in it. We had reached agreement with the applicant on the weights for the two stages and the analyses. And there weren't many dropouts in stage one of the studies. So we were able to overcome two of the big hurdles for this design in this program.
But if we receive another application with SPCD in the future, we're going to have to look at those issues again because they really are trial specific. So we'd advise sponsors to use consistent stage lengths and to reach agreement with us in advance on the primary endpoint and other critical trial features. And even if we reach agreement on all of those things, we're still not going to be able to agree a priori that the study will be acceptable because of some things that we're concerned about will remain open questions until we have that data in hand.
I already mentioned that here there weren't many dropouts in stage one. You don't know that until stage one is done. So even if we do accept the design and the study is positive and all of these issues are resolved labeling is still going to be super complicated if you have an SPCD.
[AUDIO INTERRUPTION] end up writing a label for this one.
All right. So moving from complicated to something much more straightforward. This is a table taken from the clinical study section of the valbenazine label. This is the data that supported the approval of valbenazine for the treatment of tardive dyskinesia. The studies that supported this application really provide a good example of one of the strategies to mitigate placebo response that has been, you know, successful. And that's the use of blinded central raters.
In this study, the raters were blinded to treatment assignment and also to visit number. And using the blinded central raters was feasible here because the symptoms of tardive dyskinesia are directly observable and can even be captured on video. So they can be rated by the remote central raters fairly easily.
And then you'll note here that the change from baseline on the AIMS and the placebo arms was basically negligible.
All right. So I think it's also important to bear in mind that this phenomenon of placebo response in clinical trials is not something that's unique to psychiatry. We see it in multiple other areas of medicine. It's ultimately the reason that we have placebo controlled studies in the first place.
We do expect to see some response in a placebo group. Folks get something that they think could be an active drug and, lo and behold, they have some response. It's important, though, if you want to understand that the observed response is, in fact, related to the active treatment that you do show that folks on the investigational drug are doing better than folks on the placebo.
So for the next couple of slides, I'm going to show some examples of what we see in other disease areas and speculate a bit on why the placebo response rate in those trials is higher or lower than what we're used to seeing.
And I'll caveat this by noting that I pulled my examples from the most recent Office of New Drugs annual report, and I haven't done a deep dive to see if other drugs behave similarly or if my speculation here bears out consistently. But with those caveats in mind, I'm also going to try to draw some parallels to circumstances in psychiatry trials.
All right. So the first example I have here is from the clinical study section of labeling for zavegepant, which is an intranasal calcitonin gene related peptide antagonist that's approved for the acute treatment of migraine with or without aura in adults.
The point I want to make with this example is that the endpoint here, pain, is very subjective. So similar to a lot of what we do in psychiatry, the endpoint is relying on patient report of their subjective experience.
Now, in this case, it probably helps somewhat to have a dichotomous endpoint of pain free versus not, rather than asking participants to rate their pain on a Likert scale that would introduce more variability. And honestly, as somebody who gets migraines, I can tell you that pain free is what matters. Like, a little bit of migraine pain is still migraine pain. Like, I don't want to deal with it.
Anyhow, with that kind of subjectivity, it's not too surprising that about 15% of the folks in the placebo group were responders.
Now, if you think back to that slide I showed earlier about contributors to the placebo response, some of this could be placebo effect. Some of it could just be that their migraines were resolving spontaneously within two hours anyways. Regardless, we have a pretty high placebo response rate here.
But we also have a responder rate of almost 24% in the active treatment group and a statistically significant difference on the primary endpoint of pain free at two hours.
On the secondary of relief from the most bothersome symptoms, so things like photophobia, phonophobia, nausea, both the placebo and the active groups had even higher response rates, but again, a significantly higher response in the active treatment group than in placebo.
So this is from the clinical pharmacology section of that same label. And I want to point out that this is very similar to what a lot of our drugs look like in psychiatry. We describe what the drug does at the receptor level, and then we say that the relationship between that action and the clinical effect on depression or schizophrenia or whatever is unknown. And until we have a better understanding of pathophysiology, that's going to continue to be our approach in labeling.
All right. The next example I have comes from the clinical study section of labeling for linaclotide oral capsules. And I have to say, when I'm talking outside of my own disease area, hopefully I'm getting these pronunciations right. But anyways, it's a guanylate cyclase C agonist. The data here supported the irritable bowel syndrome with constipation indication.
And I think this is a really interesting example because we have two different endpoints here. Like our last example, one is a pain endpoint that's likely to be highly responsive to placebo. Again, it's subjective. But unlike the last example, it's not dichotomous. So it requires a bit more interpretation.
The other endpoint is something that's a bit closer to objective. CSBM is complete spontaneous bowel movements. So, clearly, the number of bowel movements is something that can be counted. But the endpoint itself is a little bit of a hybrid because it also involves a subjective report of the sense of completeness of evacuation.
So, interestingly, you see a much higher percentage of placebo subjects meeting the criteria for responder on the fully subjective pain endpoint than you do on the CSBM endpoint.
And I got to tell you, Section 12 of this label is something that I dream about being able to do for psychiatry. We can only aspire to this, frankly, at this point. The language here very clearly lays out the pathway between the action of the drug and the downstream physiologic effects on constipation. And it even presents an animal model to support the drug's effect on pain. So this suggests that the drug acts on some aspect of the underlying pathophysiology of IBS C.
All right. So, so far I started with an example of a trial with a subjective endpoint, then went to something that's a little bit more objectively measurable. Here I'm going to show data from the bimekizumab label and the studies that supported its indication for the treatment of moderate to severe plaque psoriasis in adults.
So bimekizumab is a humanized interleukin 17A and F antagonist. The endpoints in the study were Investigator Global Assessment, which is an overall assessment of psoriasis severity, and the Psoriasis Area and Severity Index. Now, you might think that these things are somewhat subjective because they are investigator assessments and, of course, require some interpretation to get to the score on these scales.
But these are assessments of the size and extent of the psoriasis plaques, things that are directly observable. And both scales have anchors that describe what type of appearance the plaques of a given severity would have. So, you know, it kind of like gives you a framework for how to, you know, rate these different lesions.
So even though these are global assessments and you might think of clear and almost clear as being analogous to something like improved or much improved on a CGI, we're really talking about very different things.
Here, both what the patient is experiencing and what the clinician is observing are things that you can see and measure. You're not asking the patient if the patient feels like their skin is redder, you can see the erythema. And here you can see a much lower rate of placebo response in the studies. When you're directly observing the pathophysiology in question, and it's something that is objective or relatively objectively measurable, you get less placebo response.
All right. And Section 12 of this label isn't quite as definitive as the linaclotide label in terms of directly linking the drug effect to pathophysiology, but it's pretty close. And, again, it's probably a combination of the relatively objective outcome measures and the tight link between drug action and pathophysiology that's contributing to the low placebo response in these trials.
Finally, I want to put up an example that, of course, has been in the news a lot lately. This is from Section 14 of the tirzepatide label, and this is one of the GLP 1 inhibitor drugs that's indicated for chronic weight management as an adjunct to reduced calorie diet and increased physical activity.
Now, there are all sorts of things that can contribute to placebo response in weight management studies. So, for example, the folks who are in these studies are likely to be motivated to lose weight in the first place. They're required to engage in diet and exercise as part of the study. And even though it's difficult, sometimes folks just lose weight.
So even though weight is something that is objectively measurable, there's multiple physiologic and behavioral factors that may contribute to changes in weight. So there's a lot of variability, and it's been traditionally pretty difficult to show improvement in weight loss trials, or at least to show enough improvement that it overcomes the adverse events that are observed in the trials.
Anyways, the primary outcome in these studies was the percent of patients losing at least 5% of their body weight [AUDIO INTERRUPTION]. Now, you'd think that that would be pretty difficult to surpass, but these studies still managed to show a treatment difference because the active treatment works like gangbusters.
So another way to overcome concerns about placebo response is to find something that really has an impressive treatment effect. Then, even if you have a massive placebo response rate, you'll still be able to show a difference. And so far we don't have much of anything with this kind of an effect in psychiatry, unfortunately.
And then again, once again, in Section 12 we have a mechanism of action description that links the drug action directly to the clinical effects. The drug binds to a physiologic regulator of appetite, the person taking the drug eats less. It's pretty straightforward.
All right. So what lessons can we take away from all of this? Ultimately, the point that I want folks to take home from the examples I've shown in psychiatry and in other disease areas is that there are things that we can do to help mitigate the placebo response in our clinical trials. For things like SPCD or other nontraditional study design elements, I would advise sponsors to talk to us early and often. There are still some methodological issues that, you know, need to be overcome, but we're willing to consider SPCD studies as long as we're able to agree on specific aspects of the design and analysis.
Folks can also do things like trying to improve rater training and to mitigate some of the variability that's just inherent in asking human beings to assign a rating to something that is subjective.
Still related to measurement, but maybe more of a medium term than a short term solution, it could be worthwhile to develop better clinical outcome assessments. The scales that we use in clinical trials now have been around a long time. You know, they were mostly expert consensus and, you know, just they're face valid, for sure, and obviously we have precedent for them, but they've been around longer than modern psychometric principles, quite frankly. So developing new ones would potentially be welcome.
Anyways, in terms of other sources of variability, I'd refer back to Dr. Detke's presentation and his comments on the number of sites, enrollment criteria, and so on. Essentially, quality controls on study design and implementation. But ultimately what's really going to be the real game changer here is when we can develop drugs that actually target pathophysiology. That's when we'll finally be able to take some of this variability and subjectivity out of our clinical trials and really get much more objective measures.
In the best of all possible worlds, we would have a much better understanding of pathophysiology of psychiatric disorders. We'd be able to develop drugs that target the pathophysiological underpinnings of our diseases, and we would even be able to define study entry criteria more appropriately because we wouldn't be relying on subjective assessments for diagnosis or inclusion.
We'd be able to get that blood test or get that scan that can tell us that, yes, this is, in fact, what's going on here, and this is a patient who is appropriate for this clinical trial.
And I understand that we're, you know, a long way from that today, but I hope that folks will think of this as an aspirational goal, that our current state of understanding is less of a roadblock and more of a call to action.
And so with that, and recognizing that I am the one thing standing between you and our break, I will just say thank you very much for your attention.
TOR WAGER: Okay, wonderful. Thank you to all of our speakers and panelists in this first session.
Let's take a short break. We have some questions in the chat. More questions are coming in. But we have a break now until 1:50. And so I suggest that it's a short break, but we can get back on track and start then in about seven minutes. Okay? Thank you.
TOR WAGER: Okay. Hi, everybody. It's a short break, but thanks for hanging with us here and coming back after this short break.
Current State of Placebo in Device Trials
TOR WAGER: Our next session is going to be led off by Dr. Holly Lisanby and Zhi De Deng on the current state of placebo effects in device trials, and then we'll go for a series of placebo effects in psychosocial trials, and then, after that, the panel discussion. Dr. Lisanby, thank you.
Sham in device trials: Historical perspectives and lessons learned
SARAH “HOLLY” LISANBY: Thank you, Tor. And so these are my disclosures. And as Tor said, I'm going to be talking about placebo in device trials. And so although up until now in the workshop we've been talking about placebo in drug trials, which are typically given either by mouth or intravenous or intranasal, we're now turning our attention to how you would do a placebo in a device trial.
And that's where we use the term sham. So we blind device trials typically by doing a sham procedure. And the idea of sham is that the mode of application of the device and the ancillary effects that the device elicits are meant to be as closely matched as possible but without having active stimulation of the body or the brain specifically.
Now, one of the challenges in blinding device trials using sham procedures is that one sham does not fit all or even most. And let me explain what I mean by that.
There are a growing range of different devices. Here you see the landscape of neuromodulation devices. On the X axis is how invasive they are and on the Y axis is how focal they are. And they all use different forms of stimulation applied to the head or the body. Some are surgically implanted, others are not. And those are just the devices that directly apply energy to the head or cranial nerves.
But there's another space of devices that deliver audio or visual stimuli to affect brain activity indirectly, and these include prescription digital therapeutics and neurofeedback devices.
Now, even within one modality of device, here I'm going to use transcranial magnetic stimulation, or TMS, as an example. We have a broad range of different TMS devices. Here I'm showing you just a few of them. And while they all use rapidly alternating magnetic fields, they differ in how they apply that to the head.
So this device, for example, uses an iron core figure 8 coil. This device uses an air core figure 8 coil. Now, those are pretty similar in terms of the electric field induced in the brain, but this device uses three different types of coil that are called H coils with different coil windings that stimulate very different parts of the brain and have different ancillary effects.
The device on the left uses an air core figure 8 coil, but it has some additional bells and whistles to it. It uses neuronavigation. So there's a camera in the room and a tracker to be able to navigate the TMS coil to a specific spot in the brain that was identified before treatment on the basis of fMRI. And so there's an additional aspect of this procedure. And also it's given with an accelerated schedule, where ten treatments are given a day, each day, for five days.
Now that brings us to some of these ancillary effects of TMS. One is the intensive provider contact in a high tech environment. And I'm showing you here just a few pictures from our lab. And this is intensive contact. It can range from either one session a day for six weeks to ten sessions a day over five days. And this really highlights the importance of blinding, not just for the patient, but also the coil operator and the raters.
Now, there are also sensory components to TMS. It makes a clicking noise, which is induced by the vibration of the coil within the casing. And this is quite loud. Even with earplugs, you can't mask the bone conduction of the sound. And so that, in addition to the sound, which can it also can induce scalp sensations. And these sensations can range from just feeling a tapping on your head to feeling something that's a scalp discomfort, even to scalp pain.
And the TMS can also evoke movements. So if you're even if you're not over the motor cortex, if you're over the frontal cortex, which is for depression treatment, this can cause movement in the face or the jaw, which can be from directly stimulating scalp muscles, facial nerves, or cranial nerves.
You can also, depending on the shape of the coil, get some evoked movement from the motor cortex. And this is more common with the more diffuse coils, such as the H coil configurations.
Now, not only are these ancillary effects important for blinding of clinical trials, they also represent important confounds for physiological studies that we do with TMS, where we want to understand use TMS to probe brain function, such as coupling TMS with EEG to study evoked potentials or coupling TMS with fMRI.
Now, sham TMS has evolved over the years. I'm showing you in the center of this photograph active TMS, and in the corners are four different types of early forms of sham TMS, which were called coil tilt TMS configurations, where you tilt the coil off the head so that the magnetic field is sort of grazing the scalp. You get some sensation, you get the noise, but you're trying to not stimulate the brain.
Now, while this coil tilt sham does induce some scalp stimulation and clicking, it lacks operator blinding. But even worse than that, what we showed from intracerebral recordings of the electric field induced in the brain by these different forms of a coil tilt sham in non human primates is that compared to active TMS, which is the top line, one of these four sham coil tilt configurations was almost 75% strength of active TMS. And that's the second line from the top with the black circles.
And so some forms of these coil tilt shams were actually biologically active. And that represents a confound when you're trying to study the older literature, trying to look at, do meta analyses of TMS clinical effects.
The next evolution in the step of sham TMS was shielding. And for example, figure 8 coils could have a metal shield between the coil and the head that blocked the flow of the magnetic field. And here, this E shield has both the magnetic shield as well as a printed circuit board on top of the coil that was meant to be fired antiphase with the TMS in order to try to cancel out the magnetic field at the surface of the head.
These types of approaches look and sound like active TMS, and they provide operator masking. However and they're biologically inactive. However, they don't feel like active TMS. Here you're looking at subjective ratings of scalp pain, muscle twitch, and facial pain with active TMS in the red and sham in the black. So there's not appropriate masking or matching of these ancillary effects.
But that sham, the E shield sham was used in the pivotal trial for depression in adults. And that pivotal trial missed its primary endpoint, which is shown here in the yellow box, where active TMS is in the blue line and sham is in the gray line.
Ultimately, TMS became FDA cleared in 2008 for a limited indication based on this post hoc analysis, which I'm showing you here, where about half of the patients in the pivotal trial who had failed only one antidepressant medication in the current episode showed a significant separation between active in the black line and sham in the gray line. However, those who had more failed trials in the current episode, from two to four, did not separate between active and sham.
Subsequently, the label was expanded and CMS coverage determinations have been provided, but that was on the basis of additional evidence, which came from additional randomized controlled trials as well as open label experience and literature reviews.
Now, that same sham has been used in a pivotal trial for TMS for adolescent depression, which also failed its primary endpoint and failed to separate active from sham. Here you see the antidepressant scores on the Y axis with active TMS in the blue and sham in the red, and they were indistinguishable.
And the sham is described in the paper, as I'm showing you here in the quote, and this is another one of these metal shield or E shield shams that did not provide scalp stimulation.
Now, ultimately, FDA did clear TMS down to the age of 15 on the basis of retrospective analysis of real world data that were derived from a registry of over a thousand adolescents over a span of 15 years, all of whom were obviously receiving off label treatment, as well as a literature review. And the status of insurance coverage is to be determined.
The next step in the evolution of sham TMS was scalp stimulation, and that's what we used in the OPT TMS trial of almost 200 patients. And this was the first study to use scalp stimulation. And you see those little patches on her forehead. Those are electrodes through which we administered weak electrical stimulation to the scalp along with auditory masking in order to better mimic the ancillary effects of TMS.
And here you can see the ratings of scalp discomfort and headache were similar between active TMS in the red and this scalp stimulation sham in the black.
This, we did assess the integrity of the blind in the OPT TMS trial, and we found that the blind was preserved, very low percentage of extremely confident correct responses. And we found a separation between active and sham in this study with a 14% remission with active and 5% remission with sham. That was statistically significant.
Shams in the modern era have kept this idea of scalp stimulation and auditory masking, but they come in different versions that are now available as turnkey systems. For example, this sham, which has an active magnetic stimulation on one side of the coil and no stimulation on the other side, but the sides are identical in appearance, and this comes along with an adjustable output for electrical stimulation of the scalp, which is synchronous with the TMS pulses that's built into the system.
Now I'm going to shift from TMS to a different form of stimulation, transcranial direct current stimulation, or tDCS. This is from one of the randomized controlled trials that we conducted of active versus sham tDCS for depression in 130 patients, which failed its primary endpoint.
Now, I'm showing you the depression response on the Y axis for unipolar patients on the left and bipolar patients on the right. And although we did not find active tDCS to be better than sham, we found something curious, which was that sham was better than active, particularly in the unipolar patients. And that caused us to ask, well, what is going on in our sham tDCS intervention?
Here's what our active intervention looked like. We stimulated at 2.5 milliamps continuously over 30 minutes. The sham, which we thought was biologically innocuous, actually had these brief ramp ups and then ramp downs intermittently during the 30 minutes.
But in addition to that, it had a weak current of .032 milliamps that was continuous throughout the stimulation. We weren't aware of this continuous stimulation, and it begs the question whether this waveform might have had some biological activity. And certainly when you find sham better than active, one has to ask that question.
Now, this question of how to sham tDCS trials has been addressed in the literature. In this study in 2019, they reported that there were a great multiplicity of sham approaches that were being used in the field. And some of these might have biological action.
Now, in 2018 we had conducted an NIMH sponsored workshop and published a report from that workshop in which we urged the field to present the rationale and the effectiveness of sham stimulation when you do studies. And we observed that this is rarely documented. We also encouraged the field to do blinding checklists during the study design, reporting, and assessment of study validity. And we still encourage this. It's still timely.
Now I'm going to move from tDCS to another form of implanted stimulation. So TMS and tDCS are non surgical. Now we're dealing with a surgical implanted device, vagus nerve stimulation.
So it's surgically implanted pulse generator, and sham is done by implanting the device but not turning it on. The pivotal trial of VNS for depression failed its primary endpoint, which is shown in the yellow box here. But it was subsequently FDA cleared based on a non randomized open label comparison with treatment as usual, as you see here. Insurance coverage was frequently denied, which limited utilization.
More recently, there was a study called the RECOVER trial, which stands for randomized controlled blinded trial, to demonstrate the safety and effectiveness of VNS as an adjunctive therapy versus no stimulation control.
This RECOVER study was designed in accordance with the CMS coverage with evidence determination decision memo. The study is not yet published, to my knowledge, but according to a press release from the company that sponsored it, after one year of active VNS versus sham, which was implantation but not being turned on, this study failed its primary endpoint.
And I'm quoting here from the press release that it failed due to a strong response in the sham group, which they said was unforeseen in the study design. And I would say that we might have foreseen this based on the original pivotal trial, which also failed to differentiate active versus sham.
Now I'm going to move to deep brain stimulation. And this is the randomized controlled trial that we conducted on bilateral subcallosal cingulate DBS for depression. Sham was done by implanting but not turning it on. And this study, in a futility analysis, failed to differentiate between active and sham. So you can see this has been a recurring theme in the studies that I've shown you.
Now, there's some specific challenges to blinding DBS trials. By the time you get to DBS, you're dealing with a very severely ill, depressed population, and clinical severity may represent some dangers when you try to think about the relapse that may occur from crossover designs, like crossing over from active to sham.
There are unique things that may unblind the study such as battery recharging or batteries that don't need to be recharged that could cue a patient. And also there's a need for rigorous safety protocols to protect patients who are so severely ill during their sham phases due to the risk of clinical worsening.
So, to conclude, sham methodology poses a lot of complex challenges for device trials. One size does not fit all. The interpretation of the literature is complicated by this variability in the sham methodology across studies and across time as the sham approaches have evolved.
Measuring the biological activity of the sham intervention before using it in a clinical trial is important and it is seldom done. And assessing the integrity of the blind is important for patients, operators, and raters. And that's why with sham procedures we need to think about triple blinding, not just double blinding.
And the shortest pathway to regulatory approval, which I gave you in the example of VNS, does not guarantee insurance coverage nor clinical adoption.
Some thoughts about future directions. We could focus on developing next generation active devices that lack these ancillary effects that need to be mimicked by sham. Some examples that you'll hear about from Zhi Deng, who's coming up next, include quiet TMS and controllable pulse TMS. We could conduct studies to validate and characterize the biological actions and expectancy effects of sham interventions. And there's a role for active stimulation of a control brain area as a comparison condition.
These are the members of the Noninvasive Neuromodulation Unit in our lab at NIMH. And I'll just show you the slide that we're recruiting for jobs as well as for patients in our trial. And thank you very much, and let me hand it back to you, Tor.
TOR WAGER: Wonderful. Thank you, Holly. All right. I think we have Zhi up next. So please take it away, Zhi.
Challenges and Strategies in Implementing Effective Sham Stimulation for Noninvasive Brain Stimulation Trials
ZHI DE DENG: I will share screen and maximize it. Good day, everyone. Thanks for having me here today. And for the next few minutes, I will discuss the challenges and strategies in implementing effective sham stimulation for noninvasive brain stimulation trials.
Dr. Lisanby has already gave a very nice overview as to why this topic is crucial as we strive to improve the validity and reliability of our neurostimulation device trials. I'll be discussing in more in depth the physical characterizations, computational modeling, as well as some measurements that we took of various sham strategies and discuss their trade offs in case you are interested in picking or implementing a sham technique or improving one. And I'll be focusing primarily on TMS and tDCS.
Before we proceed, I need to disclose that I am inventor on patents and patent applications owned by various institutions. Some of them are on brain stimulation technology. Additionally, this work is supported in part by the NIMH Intramural Research Program.
So when we talk about ... is this panel in the way? Let me put that aside.
TOR WAGER: It looks good. I don't think we can see it.
ZHI DE DENG: Okay, good. So when we talk about creating a valid sham TMS, Dr. Lisanby has already mentioned that there are several critical elements that we need to consider.
Firstly, the sham should look and sound like the active TMS to ensure a blinding. This means that the visual and auditory cues must be indistinguishable between sham and active conditions.
Secondly, the sham should reproduce the same somatic sensations, such as coil vibrations and scalp nerve and muscle activation. This sensory mimicry is essential to maintain the perception of receiving active stimulation.
And finally, perhaps the more important one, that there should be no active brain stimulation, which means that the electric field induced in the brain should be minimized to avoid any therapeutic effects.
For TMS, there are several categories of ways to implement sham, which are loosely categorized into the coil tilt techniques, two coil configurations, and dedicated sham systems. I'm going to describe each of them in some detail next.
So Dr. Lisanby has already covered the coil tilt technique, and this is one that was pretty popular in the early days of TMS. By angling the coil 45 degrees or 90 degrees relative to the tangential plane of the head, one can minimize the stimulation to the brain. At least they thought so.
It turns out through modeling and also intracranial recordings of induced voltages that some of these coil tilt techniques remain biologically active. Here you see simulations on a spherical head model of various coil manipulations in coil tilt. Up here we have the active figure of 8 stimulation producing a single focus of electric field directly underneath the center of the figure of 8 coil.
When you tilt the coil 45 degrees or 90 degrees, and when you look into the brain, there is considerable residual electric field that is still induced with these coil tilt techniques.
A better way, a very clever way, and this is popularized by some folks in Europe who's doing motor excitability studies, involve two coil configurations. You use two TMS coils that are attached to two different TMS stimulators, and you would position these coils perpendicular to each other, one in the active tangential configuration and one that is 90 degrees on top of the active coil.
And with this technique, the advantage is that you can interleave active and sham TMS pulses in the same protocol because you are dealing with two different TMS stimulators. So in active mode, you would simply fire the coil that is closer to the head, which is tangential in the active configuration. In sham mode, you would simply fire the coil that is on top of the active coil.
However, this technique, like the coil tilt, there is a spacer involved in this perpendicular coil setup. So the field that is induced in the brain is less compared to the 90 degrees coil tilt, but it does also not induce any scalp stimulation. That means that the sensation at the scalp level is decreased and not felt by the participants.
Another implementation involves a sandwich design, also involving two coil setups that are sandwiching a metal shielding plate. In active stimulation mode, one would fire the coil that is closer to the head, and in sham mode one would fire the coil that's further away. And this shield ensures that you have the -- limits the penetration of the magnetic field, resulting in no scalp stimulation as well as no brain stimulation.
The final category of sham systems are these dedicated sham systems manufactured by different companies, the first of which is a reversed current sham. Magstim has an implementation of this concept. In active stimulation, the coil current in the coil is such that there is a same coil current direction underneath the center of the coil, summating the field underneath the center.
In the sham stimulation setup, the coil current in one of the loops is reversed such that at the center of the coil the field is canceled. This effectively creates a larger circular or oval type coil, which is a larger coil that has a lesser field decay, and so when you actually look into the brain, there remains substantial electric field stimulation there.
Another technique that was mentioned earlier is shielding by, again, putting a metal shield or new metal shield underneath the coil. You can effectively block out all of the field penetration, but one would also completely eliminate any scalp stimulation, making the sensation feel different.
Another implementation strategy involves using a spacer and a passive shielding. This is an implementation of the MagVenture coil, for example, using a large block coil, and the coil winding inside that large block is only built into one side of the coil. And so during active stimulation, one would flip the coil such that the active winding is closer to the head. And for sham stimulation, one would flip this coil over such that the passive shielding is closer to the head and the active winding elements are further away from the head.
This shield technique plus the spacer would completely eliminate any brain stimulation, but it also would eliminate any scalp stimulation.
A final coil setup was invented by our lab several years ago, which we called the quadrupole coil. This implementation splits the figure of 8 coil into four loops, and by reversing the coil current direction on the outside loops during sham stimulation, effectively, you may get into a smaller figure of 8 coil. And as we know, with smaller coils, it has a lower field penetration, and therefore the scalp stimulation is reduced as well as the brain stimulation is reduced.
How do all of these different sham stimulation strategies stack up on each other? The criteria we want to achieve is basically 100% scalp stimulation compared to the active electric field. So when we quantify this sham electric field at the scalp, one would like to achieve 100% compared to the active E field in the active configuration.
When it comes to brain stimulation, in sham, E field should be zero. You don't want any electric field induced in the sham condition. And so one would like to maximize this contrast between scalp stimulation and brain stimulation.
But looking across the coil tilt techniques, the two coil configurations and dedicated sham systems, none of these techniques perfectly achieve what we want. Either you have no scalp stimulation, but it also has no brain stimulation, or you have residual scalp stimulation and brain stimulation at the same time, confounding clinical trial results.
So these are the primary challenges in implementing sham systems. There is a incomplete mimicry of sensory experience that is the scalp stimulation or that you have too much of this residual, possibly biologically active brain electric field that is induced.
So why don't we take a coil that does not produce any brain stimulation and produce no scalp stimulation and add to it some scalp stimulation back? And this is a proposed technique using concurrent cutaneous electrical stimulation, which was used in some of the early clinical trials of TMS, utilizing two electrodes that are placed relatively close together, approximately one centimeter's edge to edge distance underneath the center of the coil.
And the placement of the electrodes is such that you maintain the current direction induced in the head compared to active TMS. And the current is mostly shunted in the scalp, but a little of it enters the brain.
The early implementations of this technique would use a customized ECT device, and the device would deliver low amplitude square pulses that are synchronized to TMS pulses. In more modern configurations, this electrical stimulation module is incorporated into a dedicated sham coil, for example, such as the MagVenture setup.
There are several ways to use this electrical stimulation. One way is to carefully titrate the stimulus intensity for this electrical stimulation to match the active TMS sensation, or some labs maximize the intensity of the electrical stimulation, and this electrical stimulation would be delivered in both active and sham TMS conditions to entirely mask scalp sensation in both conditions.
Now, there are some problems with this cutaneous electrical stimulation, the first of which is waveform considerations. What is the waveform of these electrical pulses that are accompanying this sham TMS pulses? First of all, the manufacturers specified triangular waveforms with a 200 microsecond rise time and a 2 millisecond fall time.
When we actually make measurements of these current pulses, though, the waveform deviates substantially from this triangular waveform that manufacturers specified in their manual. What we actually measured are these exponential decaying waveforms that has a much longer tail compared to the 2 millisecond fall time of the triangular waveform.
What's more is that if one were to characterize the decay constant of this exponential decay and plot it as a function of the intensity of these pulses, one would find that for pulses that are more intense, you have a shorter decaying constant, and therefore it's more pulsatile. If you reduce the electrical intensity, you would end up with a pulse waveform that is longer and longer. And I'll tell you why that's important a little bit later.
A second feature that is peculiar of this system is that the current amplitude is not linear with the dial setting. That is, if you were to increase the intensity from rotating the dial on the machine, a increase from setting of 1 to 2 is not the same as a setting jump from 8 to a 9, for example.
And the maximum current at maximum stimulator setting is upwards of 6.7 milliamps, which is considerably higher compared to other electrical stimulation such as tDCS, which typically uses 2 milliamps.
There's another issue with this electrical stimulation intensity, which is that this electrical E stim intensity was advertised to scale with TMS intensities. That is, as you dial up the intensity of the TMS pulses, the intensity of the electrical stimulation should also increase.
And this is not the case from our measurement. As you can see here, at two different electrical stimulation intensity settings, as we dial the TMS pulse intensity up from 50% to 90%, the amplitude of these electrical stimulation waveforms, they don't really change.
Why is pulse shape matter? Why do pulse shape matter? This has to do with the strength duration property of the sensory fibers underneath the TMS coil. Sensory fibers are classified in this rudimentary drawing of sensory nerves that I put up here.
There are A beta nerves, which are these larger diameter myelinated nerves. And typically they have faster conduction time, and so they carry information about vibrations, pressures, and touch. A delta nerves are slightly smaller, about one to five microns in diameter, and they typically carry information about sharper pain. And then we have these C fibers that are unmyelinated and they are smaller in diameter. And because of the lower conduction time, they would carry information about burning sensations and thermal pain.
I know this is not a very professional drawing of these nerves, and, of course, when it comes to drawing, I am no Rembrandt, but neither was Picasso.
This is actually a more professional drawing, but the important thing about the different pulse shape is that they preferentially activate different kinds of fibers with different time constants. So one can actually model that using a nerve model, which I have done here, and we can show that the proportional nerve activation is different across different waveforms.
On the left cluster of bars, we see what the profile of the proportional nerve activation is like for various types of TMS waveforms, including biphasic sinusoids, monophasic sinusoids, and controllable pulse width, which are near rectangular pulses.
These TMS waveforms preferentially activate A beta and A delta fibers, contributing to this tapping sensation that you feel with TMS.
But when it comes to electrical stimulation using these exponential decaying waveforms, you see that these waveforms preferentially activate C fibers. Not only that, as you change the intensity of the stimulation from maximum to minimum, you preferentially stimulate more and more of the C fibers. That is, if you decrease the amplitude, the tail here gets longer and longer, and you stimulate more and more of these C fibers, and you create more and more burning sensation and this tingling sensation that sometimes people report with tDCS, for example, which is uncomfortable to some people.
But as you increase the electrical stimulation intensity, yes, the pulses become shorter and it feels more pulsatile, but then the intensity is increased, so now it feels more painful.
And so that does not seem to be a way to achieve a very comfortable setup with this electrical stimulation. And what's more important is it does not feel like TMS, that the profile of these nerve activation is very different from a TMS waveform.
So we did not find any perfect sham. The next order of business is that we look into the clinical literature. Might there be any other stimulation parameters such as intensity or stimulation site or stimulation protocol that are predictive of sham response, something that we can modulate and modify.
So we looked into the literature, and we replicated and extended a previous meta analysis looking at depression trials that are randomized controlled trials of TMS. The average sample size across these trials are 35 subjects. In terms of stimulation protocol, predominantly high frequency stimulation and the second largest group would be low frequency stimulation.
In terms of intensity, we have a mixture of intensity with most protocols administering either 100%, 110% or 120% of motor thresholds. In terms of stimulation site, most of these clinical trials use left dorsolateral prefrontal cortex as the treatment target. That as a single site stimulation combined with bilateral dlPFC account for close to 80% of the clinical trials.
In terms of targeting approach, I was surprised to find that we were still using the scalp based targeting strategy of the five centimeter rule, which uses just measurements on the scalp, five centimeters on the scalp anterior to the motor hotspot. And that's where they determine the location for the left dorsolateral prefrontal cortex.
In terms of sham type, a lot of the earlier studies, as Dr. Lisanby mentioned, uses the coil tilt configuration, either 45 degrees or 90 degrees. And so in this analysis, they still account for majority of the studies, and only about a third of the studies included uses a dedicated sham's coil setup.
Manufacturers, you know, it's a mix. In terms of coil types, they're predominantly a figure of 8 coils. And in terms of the number of sessions that are in these studies, the median is 12 sessions of treatment.
So what did we find? What are the correlates of sham response in these clinical trials? The first thing we found was that the number of sessions is correlated with sham response. So here on the Y axis, we're plotting the percent change from baseline for the primary outcome of the study, typically a depression severity rating. So down is actually good, antidepressant. And here we see a weak correlation between the number of sessions in a typical clinical trial with improved sham stimulation.
And this, you know, over a longer treatment course, participants may develop stronger expectation of improvement, and this continued engagement with the treatment process plus regular clinic visits and interaction with a healthcare team can reinforce these expectations contributing to this sustained and enhanced placebo response, which can also accumulate over time.
The second correlate that we found to be significantly correlated with sham response is active response. So in any given clinical trial, the higher the active response, the higher the sham response. And the correlation between sham and active responses may indicate that the mechanisms driving the placebo effect are also at play in the active treatment response.
This correlation might also reflect any form of intervention. And this finding underscores the importance of effective blinding and management of participants expectations and in account for placebo effects in clinical trial design and interpretation. And the final correlate is effect of time. Something that was also mentioned in relation to pain medication a little bit earlier. So Dr. Wager mentioned earlier sham response seems to be increasing over time. We also observe this effect.
Now this increase in placebo response with drugs is sometimes hypothesized to be associated with societal changes in the attitude towards certain types of treatments and perhaps greater awareness in medical research and increased exposure to healthcare information. And also more advertising in general, particularly post approval of a drug or a device. And all together it can enhance participants' expectations and belief in the efficacy of certain types of treatments contributing to stronger placebo response.
Here we see the same thing with devices. There are also other interpretations of this increased placebo response. Perhaps the demographics of the characteristics of the participants in clinical trials might have changed over time. Perhaps participants today are more health conscious, they are more proactive and engage in healthcare, leading to stronger expectations of treatment options.
It could also be that sham response -- sham devices and procedures are becoming more realistic. Changing from the earlier coil till techniques and to now more dedicated sham systems that can enhance the belief that one is receiving an active treatment. The good news, though, is that active response is also increasing, although not quite at the same rate. Active response may be increasing over the years as well, likely attributed to improvements in dosing and targeting techniques.
Speaking of similarities between drugs and devices and their placebo response, there are also some key differences. A study was published last year in Neuromodulation pointing out the differential placebo responses between neurostimulation techniques and pharmacotherapy in late life depression. The time course of this sham placebo response is different between sham RTMS and placebo pills. Specifically at the four-week time point, participants receiving sham RTMS showed a significantly greater reduction in their Hamilton Depression Rating Scale compared to those receiving placebo pills. And this suggest a stronger early placebo response to neurostimulation compared to pharmacotherapy.
But when we look at 12 weeks, the placebo response for drugs start to catch up. And by the end of the twelve -- at the end of the trial at 12 weeks there are no significant statistical difference between the placebo pill response and the sham TMS response. This is important to consider if we're designing clinical trials to compare drugs versus devices, for example.
So we must take care of -- think about when to assess primary outcome and also employ statistical techniques to account for this time-dependent placebo effect.
Touching on TDCS for a second. We don't really have a lot of work on TDCS. Typical sham protocols in TDCS is implemented by changing the time, the temporal waveform of the stimulation, by ramping up during the beginning phase of the stimulation, and sometimes a ramp up/ramp down towards the end of the stimulation to give a transient sense of the brain is being stimulated. There are some protocols that maintained a constant low intensity as shown in Dr. Lisanby's slides that are these microamp stimulation which may or may not be biologically active and that may confound results of clinical trials.
NIMH Staff: Dr. De, I'm sorry, but we are going to need to wrap up to give enough time for our following speakers.
ZHI-DE DENG: Okay, wrap up. Sure. Sure. Final slides. And we're just going to be talking about the -- some of the determinants of sham response in TDCS trials. There seems to be a large sham effect. And there are some protocols that has better blinding compared to the others. And there are certain electrode placement that has lower sham response and that again, similar to TMS, the sham response in TDCS is correlated with the active TDCS response.
With that, I think I will skip the rest of this talk and, you know, allow questions if you have any.
TOR WAGER: Okay. Thank you. Great. Well, keep putting the questions in the chat. And for our panelists, please keep answering them as you can.
We'll move on to the next session right now which is going to cover placebo effects in psychosocial trials and interpersonal interactions.
So our two speakers are Winfried Rief and Lauren Atlas. I believe, Winfried, you are going to go first so please take it away.
Current State of Placebo in Psychosocial Trials
WINFRIED RIEF: Thank you. First, greetings from Germany. And I'm pleased to be invited to this exciting conference.
I was asked to talk about placebo effects in psychosocial trials. And this is certainly a quite critical question whether we can really apply the placebo construct to treatments on psychological therapies and trials in psychological therapies.
So I want to just try to highlight why this is complicated to transfer this concept to psychological treatments. But then I will dive into details how placebo mechanisms might apply and how we might be able to control them in psychological treatments.
So what is the problem? The problem is about the definition of psychological treatments. They're designed studies that utilize psychological mechanisms to treat clinical conditions. But if we consider the definition of placebo effects in medicine, this is pretty similar or highly overlapping with the definition of psychological treatments themselves.
So the impact of psychological and contact factors are typically considered the placebo mechanisms in medical interventions. So we can switch to other attempts either to define placebo mechanisms. But then we need the concept of what are specific, what are unspecific mechanisms. And this is quite difficult to define if we use psychological interventions because we don't have this very clear ingredient as we have in drug trials.
And the novel definition define placebo mechanisms as mechanisms of conditioning and expectation. But this is already a definition of psychological interventions.
And, as you know, CBT started with the concept of using learning mechanisms to improve clinical conditions. So there is an overlap in the definition what placebo mechanisms are and what psychological treatments are. And therefore it's quite difficult to disentangle the effects.
To provide more insight, I reanalyzed a meta analysis of Stephen Hoffman's group on depression and anxiety trials because they only included placebo-controlled trials on psychological interventions. For some of these trials, they were able to have some placebo-proof conditions if they also integrated some psychoactive drug arms. But most of the trials used arms that used some psycho education parts, information about the control or some supported therapies which means just to reflect emotional well being and to support emotional well being.
But some other trials used interventions that are known to be effective such as interpersonal psychotherapy or cognitive restructuring or GRN therapy. So they used therapies as control conditions that are known to be effective in other conditions. And this shows how difficult it is to define what a good placebo condition is in psychological interventions.
And in this meta analysis, in the first version of it six years ago, the authors defined a good psychological placebo conditions as someone -- as a condition that used an intervention and excludes the specific factor, only including the nonspecific factors. And these mechanisms that are used in the placebo arm should have shown to be non-effective for the treatment under -- for the clinical condition under consideration. And this is already a point that will be pretty hard to define in detail if we develop placebo conditions in psychological treatments.
Another attempt, as was already mentioned by Tor, is to disentangle the variant parts of treatment outcome. And this attempt, this approach is associated with names of like Bruce Wampold or Michael Lambert and others. And I show here the results of Michael Lambert's analysis. And you see that he defines placebo effects as the mere treatment expectation effect and to declare this is about 50% and allocates other parts of the effects to other factors.
We have to be aware that this kind of variants disentangling analysis, this is just about statistical modeling. This is not about causal investigation of factors. And a second shortcoming of it is also it does not consider the actions of these factors. And therefore the insight that we get from this kind of analysis is only limited.
But coming back to psychological treatments, we can say that patient's expectations are powerful predicters of outcome, as we know from medical interventions already. Here is data from a psychological treatment study on chronic pain conditions which shows that we find response rates of 35-36%, but only if patients have positive outcome expectations before they start treatment. And those who have negative outcome expectations have much lower success rates like 15%. And the relationship between positive and more negative expectations remains stable over month and years.
So what is the major challenge if we try to define control conditions in psychological treatments? The first point is we're unable to do a real blinding of psychological treatments. At least a psychotherapist should know what he or she is doing. And the placebo group in clinical trials often are different from the active interventions in terms of credibility or as we call it of being on a treatment -- a treatment that is as credible as the active treatment is.
And for some control conditions it's even questioned whether they are kind of nocebo conditions such as standard medical care or waiting list group. If you are randomized to standard medical care or waiting list, you might be disappointed, you don't expect much improvement. While being in the national core group might be even better, you try to do some self-help strategies, for instance. And another aspect is that the nonspecific effects can sometimes switch to become specific effects depending on what your treatment is and what your treatment rationale is.
I'll show one example of one of our studies for this effect. We investigated the treatment expectations in patients undergoing heart surgery. And before they had the heart surgery, we did a few sessions to optimize treatment outcome expectations. That means outcome expectations were moved from being a noise signal of placebo effect to being the target mechanism of our intervention. Like in this case the therapist is working with a patient to develop positive outcome expectations, what happens after they manage to survive the heart surgery.
So we did that with a randomized clinical trial with an expectation optimization in the major group when compared with two control groups. And we were able to show that if we optimize treatment outcome expectations in cardiac, in heart surgery patients, these patients really did better six months after surgery. Standard medical care has little improvement. It's mainly providing survival, which is important enough, no question about that. But where the patients are really feeling better six months after surgery depends on whether they got some psychological preoperative preparation.
And we also used this approach of optimizing expectation to develop complete psychological treatment programs also for patients with depression and with other mental disorders. So let's come to the other part of the placebo mechanisms, the nocebo effect. And I would like to report about nocebo effects in psychological treatments but the major problem is side effects and other effects are only rarely assessed in psychological treatments. This is really a shortcoming.
Here is just a top ten side effects from psychological treatments. Many of them are just increasing conflicts and problems. But some are also about new symptoms that develop. And some of our other studies we even found that symptoms such as suicidal ideation are increasing sometimes for some patients in psychological treatments. So negative side effects are an issue in psychological treatments and we need to assess them and to better understand afterwards whether nocebo effects occur.
How do they develop these treatment expectations, be it either positive or negative? One major effect was already shown in many placebo trials. And that is about pretreatment experience. Here are data of about 300 former psychotherapy users who plan to attend another psychological treatment. And you can see that how much improvement patients expect mainly depends on how much improvement they experienced during the last treatment.
And the same with negative expectations and the same with side effect expectations. Of note, positive clinical outcome expectations are not correlated with negative outcome correlations. That means people can be optimistic and worry at the same time. So a critical role about patient's frequent expectations is the clinician. And we wanted to evaluate the effect of the clinician using an experimental design. Here is our clinician. I will call him Tom. Who is explaining to a critical patient whether psychological treatments can help or not.
And we wanted to modulate this situation and therefore we first brought all our participants in this situation of developing negative treatment outcome expectations. We were quite successful in establishing negative treatment outcome expectations or as you see here, reduction of positive outcome expectations. After that, Tom explained to the patient that psychological treatments are helpful for his or her condition. But Tom changed his behavior. He always used the same information. Psychological treatments are powerful to improve your clinical condition.
But he sometimes was more warm and empathetic. Sometimes he showed no signs of competence. Sometimes both. You can see that it mainly depends on these behavior patterns of the therapist whether the information that he wants to transfer really has some action. If the therapist is low in competence and low in warmth, the same information doesn't have any effect while the same information can have a very powerful effect if the therapist shows warmth and competence.
So let me conclude these few insights into our placebo research. The distinction between specific treatment mechanisms and unspecific mechanisms is less clear than in biomedical interventions. But we can still say that expectations also predict outcome in psychological and psychosocial treatments.
And main determinant of treatment expectations are pretreatment experiences, but also the clinician/patient relationship and many other factors that contribute to a development of treatment expectations. Expectations can be an unspecific factor to be controlled for, but they can also be the focus of an intervention and can really boost their treatment effects and therefore they are -- it's really valuable to focus on them.
And, unfortunately, side effect assessments are typically overseen factors in clinical trials. I'll come to this back in a moment. We want to recommend that placebo-controlled trials are needed in psychosocial intervention -- for psychosocial interventions. But it's more difficult to decide what to include into them. The major idea is to exclude the active mechanisms, but this is not that easily to be defined and therefore we need some psychological attention conditions that are credible in our controlled conditions that psychological treatments are compared with.
I would say that we need a variety of trial designs. Maybe if you start with very new interventions, it might be justifiable to start with a waiting list control group or with a standard medical care group. But if you want to learn more about the treatment, you need more control group designs. And there is not one perfect control condition, but you need variations of it. And last, not least, we have a strong emphasis on side effects and adverse events and unwanted events need to be assessed in psychological treatments as well.
Finally, let's make two comments. I think placebo-controlled investigations are developed and have to be developed to better understand the treatment mechanisms. From the patient's view, they are less important. The patients want to know whether -- what the overall efficacy is of a treatment. That means the combination of specific and unspecific effects, the overall package. And we shouldn't lose that out of mind.
And second, all these mechanisms we are talking about, they are not really to be separated one from the other, but they are typically interacting. Expectation effects are interacting with the development of side effects are interacting with the experience of improvement that can go back to the drug or to the psychological treatment.
So, so far from my side, and I'm happy to hand over to Lauren who will continue to talk about this issue.
TOR WAGER: Wonderful. Thank you, Winfried.
Now we have Lauren Atlas.
LAUREN ATLAS: Thank you. So it's really an honor to be wrapping up this first exciting day of this workshop. And to kind of I guess in a way bring you back to some of the themes that Tor highlighted in his introduction.
So I'll be talking about why I think that we as a field would benefit from taking a social neuroscience approach to placebo analgesia and placebo effects more generally. So Tor used the same figure in his introduction to the day. And I think one of the things that I really want to highlight in this is the distinction between intrapersonal factors so things like expectations, learning, history of associations with different treatments and different clinical context. And this really has kind of been the foundation of most studies of how placebo effects works -- work really because it's quite easy to manipulate things like expectations and learning in the lab and understand how those affect clinical outcomes.
But there has been far less work on the interpersonal processes that support placebo. And in some ways I'd like to say this is really where we need to be going as a field because it could be a lot easier to teach clinicians how to enhance patient outcomes rather than sort of being to fold into what a patient brings to the table. Although of course these factors interact and are both important in determining clinical outcomes.
And so the way I like to think about this interplay is really from a social affect of neuroscience standpoint. So the term social neuroscience really has come about over the past couple of decades talking about how we can use neuroscience techniques to understand emotional and interpersonal processes across a variety of domains. And where I think about this in the context of placebo is, first of all, through neuroscience techniques we can understand how placebo effects are mediated, whether that be supporting specific different types of outcomes or more general processes that shape placebo effects across domains.
From an affect and neuroscience standpoint, we can determine whether the mechanisms of different types of placebo are shared or unique. So, for instance, in the context of placebo analgesia we can ask whether placebo affects are really supported by pain-specific mechanisms or are we looking at the same mechanisms that might also be relevant in placebo effects for depression.
And then finally, from a social standpoint we can really isolate what a role is of the social context surrounding treatment. And so I a couple of years back wrote a review kind of looking at placebo effects from this social affect of neuroscience standpoint focusing on the role of expectations, affect and the social context.
Today I'd like to focus first on mechanistic work using neuroscience to understand how placebo effects are mediated. And secondly to address the role of the social context surrounding treatment. Which I think has implications not only for the study of placebo and clinical outcomes but also for reducing health disparities more generally. And I think I do want to say that I think the study of placebo can really point to all of the different features of the psychosocial context that influence clinical outcomes.
So this is why I think there is so much we can take from the study of placebo more generally. So turning first to how placebo effects are mediated. First, throughout the day we've been talking about how expectations associated with treatment outcomes can directly influence clinical outcomes in the form of placebo. And as Tor mentioned, if we not only compare treatment arms to placebo groups to isolate drug effects but instead also include natural history control groups, we can isolate placebo effects on a treatment outcome by controlling for things like regression to the mean.
Now, again this came up earlier, but a meta analysis of clinical trials that compared placebo with no treatment revealed that there was no placebo effect on binary outcomes or objective outcomes. But there was a substantial placebo effect on continuous subjective outcomes and especially in the context of pain. The others concluded that the fact that placebos had no significant effect on objective continuous outcomes suggest that reporting bias may have been a factor in the trials with subjective outcomes.
So the idea here when we talk about kind of our model of placebo, traditionally we think that things like social dynamics, psychosocial context surrounding treatment, cues associated with treatments lead to changes in one's sensory processing or one's bodily state. And based on that one makes a subjective decision about how one is feeling. For instance, a placebo effect in depression might lead to shifts in emotional processing, or a placebo effect in pain would lead to someone reporting less pain. And this is really driven by our report biases.
The idea is that rather than expectations changing that sensory processing, they affect subjective responses directly perhaps by changing our criteria in first calling something painful. So for over two decades now the field has really focused on asking to what extent are these effects mediated by changes in sensory processing?
And placebo effects in pain are a really ideal way for us to ask this question because we can objectively manipulate pain in the lab. So we can use this device called a thermode heated up to different temperatures and measure how much pain it elicits. And the targets of nociceptive signals are well studied, very well known and we know the tracks that transfer this information to the cortex.
And these can be visualized using functional magnetic resonance imaging or fMRI. So we see reliable activation in response to changes to nociceptive stimuli in a network of regions often referred to as the pain matrix including the insulate, dorsal anterior cingulate, thalamus, medial sensory cortex and brainstem and cerebellum.
Now we used machine learning to identify pattern of weights, which we call the neurologic pain signature that is sensitive and specific to pain and can reliably detect whether something is painful or not and which of two conditions is more painful. So this really provides an opportunity to ask when placebos affect pain. So, for instance, if we apply an inert topical treatment to a patient's arm before administering a noxious stimuli that they believe will reduce pain, does this pain reduction come about through changes in pain specific brain mechanisms or do we see shifts in more general mechanisms such as shifts in affect, things like emotion regulation or value-based learning? So maybe people just feel less anxious but there is nothing specifically about pain. This isn't really a problem because this would also mean that what we're learning about might transfer to other domains.
So a couple of years back nearly all labs that use this neuroimaging to study placebo analgesia in the brain combined patient level data. And what we found is that there was a reliable reduction in pain reports during fMRI scanning when people had an analgesic treatment -- or a placebo, sorry, relative to a control treatment that they didn't believe would reduce pain with a moderate to large effect size.
But there was no reliable placebo effects on the NPS. So this suggests that really we're not seeing placebo effects on this kind of best brain-based biomarker of pain. What do we see the placebo effects modulating? Oh, sorry, it's important for me to say that even though we don't see placebo effects on NPS, there are other psychological manipulations such as mindfulness cues that predict different levels of pain or administering treatments that reduce pain both when subjects know they are receiving it or when they believe they are not receiving it. And these all did affect NPS responses. So it is possible for psychological treatments to modulate the NPS, but we didn't see any placebo effect on NPS responses.
We also conducted a meta analysis of placebo analgesia looking at other published studies. And what we found is that there were reliable reductions during pain with placebo administration in the insula, thalamus and dorsal anterior cingulate. Now these regions are indeed targets of those nociceptive pathways that I mentioned. However, these regions are also activated by pretty much any salient stimulus in that MRI scanner as well as by anything involving interoception or a tension to the body.
And so I think an important point for the discussion is to what extent are these mechanisms or any of the principles we've been talking about today unique to pain or depression or any specific clinical endpoint.
When we looked for regions that showed increases with placebo, we saw increases in the ventral medial prefrontal cortex, dorsolateral prefrontal cortex and the striatum; regions that really have been implicated in domain general shifts in affect, things like emotion regulation and learning about valued outcomes.
So in this first half of my talk I demonstrated that placebo effects seem to be mediated by domain general circuits involved in salience, affective value and cognitive control. We did not see any placebo effects on the neurologic pain signature pattern. And this really points to the idea that these placebo mechanisms are unlikely to be specific to pain.
However, you know, there is many different labs working on different mechanisms of placebo. And so I think this is an ongoing question that really demands on further trials and different comparisons within and across participants.
So now I'd like to turn to the second half of my talk addressing the role of the social context surrounding treatment. And I'm going to talk about this in terms of patient's expectations, providers' assessments of patient's pain, and patient pain outcomes themselves.
So we were interested in asking whether patient's perceptions of providers impact pain expectations. And we know from work that Winfried and many others have conducted that indeed placebo responses depend on many different factors in the patient-provider relationship including how a provider treats a patient.
So Ted Kaptchuk and his group showed that a warm provider can lead to reductions in IBS in an open label placebo trial. We just heard data on how a provider's warmth and competence can influence outcomes. And this has also been shown in an experimental context by Ally Klem’s lab. And finally -- and I'll present this briefly at the end of my talk – we also know that a patient's perceived similarity to their provider also influences pain and placebo effects in simulated clinical interactions.
So a former post doc in my lab, Liz Nekka, was interested in studying this by asking not only whether interactions between patient and provider influence pain expectations but also whether our first impressions of our providers, namely in terms of their competence and/or similarity to us influence expectations even without actual interactions.
And the reason Liz wanted to do this is because we know from social psychology that people's first impressions are really important for a lot of different behaviors. So simply looking at people's faces can predict -- and judging competence can predict the outcomes of elections. And this is work that really has been led by Alex Todorov and his group.
So these faces are morphed along a dimension of competence. And so you can kind of see moving from three standard deviations below the mean to three standard deviations above the mean that there are certain features that are sort of associated with competence and dominance and that we use to make judgments about that person's trait. And so Liz asked whether these types of first impressions also influenced expectations about pain and treatment outcomes.
We conducted five studies on -- using Amazon's Mechanical Turk. And the first studies used those morphed faces from Todorov's group. Importantly, these were just male faces in the first two studies. In our third study, we used the same competence dimensions morphed onto either male or female faces.
We conducted another study in which we removed any cues like hair or clothing and just showed the face, the morphed male or female face itself between subjects.
And in the final study we used real individual faces that varied in race and ethnicity and again had between groups a manipulation of sex. On each trial participants first went through a series of trials in which they saw two faces that varied in competence and told us which provider they would prefer for a potential painful medical intervention. And then they were asked to imagine that provider were performing a painful medical procedure on them, how painful would the procedure be. And after the procedure are you more likely to use over the counter or prescription medication assuming that if the procedure is less painful they would assume -- they would expect to be more likely to use over-the-counter medication.
We also asked about similarities, but I won't be focusing on that today. So across all of the studies, so this is chance. This is that first decision, how likely are you to select a more competent face. What we found is that participants chose the more competent looking provider based on those facial features in the first study. We replicated that in the second study. In the third study we found no difference as a function of the features related to competence. In part because people preferred doctors who -- female doctors who looked less competent based on these features.
In the fourth study we used other individual’s ratings of perceived competence and again found that people selected more competent faces. But they also preferred this particularly only in the male faces. And when we used these real individuals, we again found that other people's ratings of competence predicted somebody's likelihood of selecting that person as their provider. And this was strongest when it came to white providers. We found that competence directly influenced pain expectations in all of the studies except for study three. So here this is the association between ratings of competence and pain. And so you see higher competence is associated with less pain across all the studies but study three. And, again, all the studies showed that the stronger the competence, the more likely somebody was to say they would have an over-the-counter prescription treatment in that study. But we found an interaction with sex such that competence predicted over-the-counter treatment only for male participants whereas competent female providers were associated with higher likelihood of having prescription medication rather than over the counter.
Finally, we found that stereotypes for these kind of information about race, ethnicity and gender which we were able to test in the fifth study also impacted pain expectations. So in study five, we found that expectations about pain varied as a function of provider race. We found that people expected the least amount of pain and highest likelihood of over-the-counter medication from the Asian providers relative to all others. And we also found sex differences in the expected medication use.
And finally, when we ran the meta analysis across all the studies, we found that effects of similarity unexpected analgesic use were strongest in white participants. And this is likely to be kind of an end group preference mainly because studies one through four all included white providers. And we found no other effects of the perceived demographics themselves.
Just with the last like three minutes or so. We know that not only do patients' stereotypes impact perceptions of providers, but we also know through studies on health disparities that providers' beliefs also impact assessment of patient's pain. So Peter Mende-Siedlecki who in this area ran beautiful studies looking at how race bias on pain assessment may be mediated through perceptual changes. Peter had black or white male actors depict pain or neutral faces. And he created morphed images ranging from neutral to painful.
And what he found is that white perceivers needed more evidence of a pain expression before labeling pain on black faces relative to white faces. And the more of the difference they had in terms of likelihood of seeing pain on white relative to black faces also predicted prescribing more analgesics to white relative to black targets across a number of studies.
We asked whether we saw similar biases in evaluations of real pain by measuring facial reactions in acute pain in 100 healthy individuals who label rated pain in response to heat, shock or cold water bath. What you can see is people have very different reactions to pain. This is all kind of the same level of pain. But you see differences in expressiveness.
And we're going to be creating a public database that will be available for other researchers to use to study pain assessment in diverse individuals. We had other healthy volunteers view these videos and assess pain. And critically we selected pain so that there were no differences across target race or gender in terms of the pain or its intensity. All the videos we presented were matched. Subjects saw videos and rated whether the target was in pain or not and how intense the pain was.
And what we found is that perceivers were less likely to ascribe pain to black individuals relative to white individuals. So again, black is here in cyan and white is in pink. And the women are with the hash lines and males are solid. And these are all again selected for trials where everybody is feeling the same amount of pain. And this is really driven by a failure to ascribe pain to black male participants when they were experiencing pain. And this was supported by signal detection analysis. We found that these race-based differences in pain assessment correlated with scores on a modern racism scale but did not vary dependent on perceiver race or gender. And we're now doing a study basically looking at how this type of bias might be reduced through learning and instructions. So basically we find that when people are told about a participant's pain after every trial, they are more accurate in judging other people's pain and that whether or not people receive feedback on pain assessment accuracy improves over time as people practice, suggesting we may be able to reduce these pain assessment biases through training and perhaps in clinical samples.
And finally, I just want to acknowledge that in this kind of dyadic interaction, we really ultimately also want to look at the direct interpersonal interactions that shape placebo analgesia. And this has been done by a series of studies of simulated clinical interactions where healthy volunteers are randomly assigned to act as doctor or patient and they administer a placebo to somebody else.
So Andy Chen Chang showed that telling a doctor that a treatment was analgesic affected the patient's pain, and that this was likely to be mediated through nonverbal communication. Liz Losen's lab showed that -- or Liz Losen when she was in Tor's lab showed that the more similarity or trust somebody had for a clinician the lowest pain they experienced. And finally, Steve Anderson, a grad student with Liz Losen showed that racial concordance between the patient and the provider in a placebo context could reduce pain, particularly in black individuals. And this was also associated with reduced physiological outcome.
So just to summarize the second part on the role of the social context surrounding treatment. I've shown you that first impressions shape pain expectations. Stereotypes impact pain expectations and pain assessment. And that concordance can enhance treatment outcomes.
Finally, just to kind of make clear where I think the path forward is from this kind of social affect of neuroscience approach, I believe that further research on how social factors shape clinical outcomes including placebo effects in placebo analgesia can help us improve patient provider interactions, reduce health disparities in general and maximize beneficial patient outcomes. And that we need more work distinguishing between domain specific and domain general mechanisms of placebo in order to isolate general effects of the clinical context versus targeting disease-specific endpoints. And identifying these kind of domain-specific mechanisms and the features of both patients and providers can really help us address the goals of personalized medicine.
So with that, I want to thank the organizers again for the opportunity to present our work. And acknowledge my former post doc, Liz Netfek, my former PhD student, Troy Duline, my current post doc Allie Jao, and mention that we have positions available in my lab. Thank you.
TOR WAGER: All right. Wonderful. Thank you, Lauren. So that concludes the series of presentations for this webinar for today. But we're not done yet.
Now we're moving into a phase where we have a panel discussion. And so it's going to be very exciting. And we'll get a chance to sort of talk about some of your comments you brought up and other things.
So this is moderated by Carolyn Rodriguez and Alexander Talkovsky. So hi, thank you for doing this, and please lead us off.
Panel Discussion
CAROLYN RODRIGUEZ: Yeah, definitely. So it's my pleasure to do this with Alex. My name is Carolyn Rodriguez. I'm a professor at Stanford. And I see there has been a very lively Q&A already, and some of them are being answered. So maybe we'll just popcorn a little bit.
There is one question here which, you know, I think gets at what we have been presenting is a lot of human data. And so maybe it's just worth noting, are studies in animals free of placebo effect? And, Tor, I see you are typing an answer, but I don't know if you wanted to answer that.
TOR WAGER: Sure. Yeah, I just finished typing my answer. But yeah, it's a good discussion point.
I mean I think that one of the first studies of placebo effects was by Hernstein in 1965 in Science called Placebo Effects in the Rat I think it was called. And there's a resurgence, too, of modern neuroscience work on placebo effects in animals. Greg Corder is going to give a talk on this tomorrow as one of the group of investigators doing this.
So long story short, I think that there are conditioned or learned placebo effects. So pharmacological conditioning pairing with a drug cue or conditioning with place cues can change the response patterns of animals as well.
It's difficult to know what animals are expecting. But there is quite a bit of circumstantial evidence or other evidence from other places even from Robert Rescorla years back or from Jeff Schoenbaum that really used clever paradigms to suggest that animals, it's really a lot about the information value and that they are sort of expecting, you know, and predicting a lot more than we might at first assume.
So even in those conditioning paradigms there might be a lot of something very similar to what we call sort of internal or mental model or expectations that are -- that's happening. So that is my first -- others can jump in here and say more.
CAROLYN RODRIGUEZ: Thank you. Yeah, any other panelists -- panelists, feel free to just turn on your videos and we'll be sort of, you know, asking, anybody else want to weigh in on animals and placebo?
Go ahead, Dr. Atlas.
LAUREN ATLAS: I'd be happy to do so. So actually, there is a study I love from a former post doc who worked with me, Anza Lee, during her PhD that -- we haven't really talked about the roles of dopamine and opioids so far today, which is interesting because those often dominate our conversations about mechanisms of placebo. But Anza had a really lovely study in which she showed that dopamine was necessary for learning the association between a context and pain relief while opioids medullary receptor system was necessary for actually experiencing that pain relief. And so that is a really nice kind of disassociation between that learning development of expectation and the actual pain modulation.
So that was a really lovely place where I thought that the preclinical work had some really nice findings for those of us who are doing human studies.
CAROLYN RODRIGUEZ: Wonderful. Thank you. And I think there is still a day two, so stay tuned. There's -- I can see in the agenda there will be more on this.
But a question I think specifically for you was how does Naloxone influence the NPS? So if there's any -- I think you answered it, but if there's any additional things.
LAUREN ATLAS: I think that's a great question. And I actually don't know of any studies that have administered Naloxone and looked at NPS responses.
The Naloxone effects on fMRI responses in placebo, actually I think we may have -- I'll just say a bit of a final jury problem there. There are a lot of studies that haven't found effects. We really need everybody to kind of publish their data.
But I think we've shown that there are studies of opioid -- or there are effects of opioid analgesics. But I don't think we know anything about blocking the opioid system and its effect on the NPS. But that would be really interesting and important so that's a great suggestion and question.
CAROLYN RODRIGUEZ: Yeah, I look forward to it. That's a very, very exciting question.
I'm going to hop over to neuromodulation. Dr. Lisanby and Dr. Deng, I think you guys have already answered a question which I found fascinating about whether when you try and get the motor threshold, what -- like does that unblind people? So I loved your answer and I just wanted you guys to just say it out loud.
SARAH “HOLLLY” LISANBY: Yeah, thank you. I can start. And Zhi might want to comment as well. So as you may know, we individualized the intensity of transcranial magnetic stimulation by determining the motor threshold where we stimulate with single magnetic pulses over the primary motor cortex and measure a muscle twitch in the hand.
And this is real TMS. And we do real TMS for motor threshold determination regardless of whether the person is going to be getting active or sham in order to give them the same level of intensity and so on. And you might think, plausibly speaking, that this might unblind them if then you give them sham RTMS with repetitive pulses. It turns out that single pulses do not cause the same amount of scalp pain or discomfort that repetitive trains of stimulation can cause.
Also, the motor cortex is farther away from the facial muscles and facial nerves so there is less of a noxious effect of stimulating over the motor cortex. And because of these differences it is very -- a common occurrence that people think they are getting active RTMS even when they are assigned to sham.
Maybe Zhi may want to comment.
ZHI-DE DENG: No, I totally agree with that. The different protocols feel very different. So being non-naive to one protocol might not necessarily mean that you break a blind.
CAROLYN RODRIGUEZ: Wonderful, thank you so much. Dr. Deng, always appreciate your humor in your presentations so thank you for that.
We're going to move over -- Dr. Detke, I think you had messaged that you have a couple of slides that may address some of the questions. And particularly Steve Brennan had asked a question about COVID interference. And there was a question about excluding sites with unusual response patterns. So would love to hear more about that.
I think you are on mute, though. We'd love to hear you.
MICHAEL DETKE: There we go. I have one kind of interesting slide on COVID. It kind of doesn't -- it doesn't get directly at the placebo response.
Let me walk you through. It's a weird slide. Because we've been looking at slides all day that like from left to right is changing -- is the duration of the study or the treatment.
This is actually as you can see on the X axis is actual calendar months. And then focus at first on the blue line. The blue line is the ADCS-ADL which is a scale of activities of daily living. And there are actually questions in it about, you know, have you gone to the grocery store recently? Are you able to do that by yourself? Have you gone to, you know, attended doctor's appointments, things like that.
And the reduction from the -- from early 2020 to kind of the peak of the pandemic, this change of like five points or so, this would be kind of the biggest -- this is an Alzheimer's study -- and this would be the biggest drug effect in the history of Alzheimer's. And this changed back even faster of a similar actually slightly larger magnitude. It was also a huge change.
This is pooled drug and placebo patients. So there is nothing in here that tells you about drug effects or not. You can see this ADL was really impacted by the peak of COVID cases. And I'm actually surprised this came out as clean as it did because we had about 30% of our patients were in Europe, Italy, France, Spain. And as you may recall, the peak of cases there was at a different time than the U.S.
But I think the takeaway here is that obviously things like COVID can certainly impact assessment scales. And they are certainly going to impact scales that specifically say hey, have you gone to your doctor's office when you can't go to the doctor's office. Scales like that are going to be really more impacted obviously than, you know, maybe just -- and moods and things could be, too, obviously. That is one piece of data that I know COVID had a whopping effect on at least one scale.
As for the sites over time, there has been a lot that has been talked about and thought about, about, you know, excluding sites with high placebo response, excluding sites with low drug placebo separation. Of course, if you do that post hoc, it's certainly not valid. There's a banned pass approach where you exclude the extreme sites on both ends, high and low placebo response, is a somewhat more valid. But and my understanding from statisticians is that any of those things increase false positives if you are doing it post hoc.
The other thing to think about when you're thinking about site performance is, A, sites change over time. They have different raters, you know, that might be there for ten years or maybe ten months. And maybe the single most important point on this response is realize, you know, the average depression trial, 100 or 150 patients per arm, 80% power to see a separation. And it's really 50% power as Ni Khin has shown and others effectively.
Now imagine you are looking at a clinical trial site. They have ten patients, five per arm. What is the statistical power there? It's close to zero. And this -- so these are some data that my colleague Dave Dubroda at Lily put together a long time ago. Huge database of I think these were Prozac depression studies. And they had the same -- you know, over many studies and many of them went back to the same sites that performed well.
And as you can see here, the same slide, each chart is a site, a site that was in multiple different studies. And their performance over time and HAMD change was no different. This study is another study that just looks at these are different investigative sites in the same trial. And this is a little bit of a build, but you can see that this site and this site have virtually identical drug responses, the yellow bars. Sorry, that's supposed to be a little higher. They have almost identical efficacy response. But this one has a huge placebo response and that one has a tiny placebo response. Which is probably because they only had five or six subjects per site. And if you get just two or three huge placebo responders.
So trying to assess site performance in the context of a single trial is pretty hard just because of the Ns. And then so evaluating performance by sites is challenging. And then excluding them for reasons like high placebo responses is also challenging. So those are just a little bit of context on that.
CAROLYN RODRIGUEZ: Thank you. Yeah, appreciate that. Question for your colleague Dr. Khin, but maybe for everyone, right?
So there is a question that says isn't it difficult to say that a two-point difference on a 52-point scale is clinically significant? So I know a lot of slides we were trying to say this is going to be significant and what is the difference between, you know, these two scales. So at the end of the day we're, you know, wanting to help patients.
And so what can we say about a two-point change in significance?
NI AYE KHIN: So two-point change is the difference between drug and the placebo. So each individual might have ten-point change or 50% change depending on the individual response. And mostly drug approval is based on statistical significance.
So if there is a two-points difference between drug and placebo for, for example, Hamilton Depression Score, that's generally -- that's the approximate total point change between the two groups that most of the drugs get approved. So, of course, statistical significant changes basing -- we base for drug approval. But for in real world, we don't really know what clinically meaningful change or difference, right. So that's still an issue.
So Tiffany might be able to add more on this topic.
TIFFANY FARCHIONE: Yeah, I mean I can add a little bit. So in terms of like the depression studies, again, those were conducted before our sort of what we do now.
Like if we have a new indication, a new endpoint, something like that, we're going to ask companies to give us an a priori definition of clinically meaningful within patient change. And we're looking, like Ni said, at the difference for an individual. Not the difference between the drug and placebo. But what matters to patients. How much change do they need to have.
And then they can have that -- they can power their study to see some amount of difference that they think matters. But ultimately we have them anchor their studies to, you know, things like global assessments of functioning. We have sponsors if they are using new endpoints do qualitative work so that we can understand what that change means on that given scale. There is a lot of additional work that goes into it now. But yeah, it's the within patient change, not the between group changes that ultimately matters the most.
CAROLYN RODRIGUEZ: Thank you so much. I felt like it was worth saying out loud. And, Dr. Farchione, I know you've done a lot of wonderful work. I heard you speak at ACNP about kind of more global measurements of functioning and really thinking about patients more globally, right. You can change a little bit on a scale, but does that translate into life functioning, work function, these are the things that we care about for our patients. So thank you both for that.
I see Dr. Rief wants to weigh in and then Dr. Lisanby.
WINFRIED RIEF: Just a small little point. The more the question has to be asked about the benefit harm ratio. And it is an important issue and very good that the question was asked. If the difference is just two points, we have to compare it with the risk and potential side effects. It's not only that we can focus on the benefits.
TIFFANY FARCHIONE: We always compare it to the risk regardless of the size of that difference.
CAROLYN RODRIGUEZ: All right. Dr. Lisanby.
SARAH “HOLLY”LISANBY: So this is an opportunity to talk about outcome measures.
CAROLYN RODRIGUEZ: Yes.
SARAH “HOLLY”LISANBY: And how sensitive they are to the intervention and also how proximal they are to the intervention with respect to mechanism. These are some points that Dr. Farchione raised in her talk as well. In psychiatry, the degree to which we can have outcome measures that are more proximal to what our intervention does to engage mechanisms, this might help us be able to measure and differentiate active treatment effects versus nonspecific placebo effects.
And this is part of the rationale of the research domain criteria or dot-research platform to try to look at domains of function. To look at them across levels of analysis and have measurements that might not just be a clinical rating scale. It might be a neurocognitive task that's related to the cognitive function that might be the target of a therapy or a physiological measure that might be an intermediate outcome measure.
So I was hoping we might generate some discussion on the panel about regulatory pathways for these other types of outcome measures and how we might think about selecting outcome measures that may be better at differentiating real treatment effects from nonspecific placebo effects.
CAROLYN RODRIGUEZ: Thank you. I see Dr. Wager, I don't know if you had something to add onto Dr. Lisanby's point or if you had a separate question.
TOR WAGER: I would like to add on to that, if I may.
CAROLYN RODRIGUEZ: Okay. Yeah, of course.
TOR WAGER: I think that's a really important question. I'd love to hear people's opinions about it. Especially the FDA, you know, Tiffany's perspective on it.
Because for me to add to that, I just was wondering how strongly the FDA considers pathophysiology in mechanism of action and what counts as mechanism of action. So there are certainly certain pharmacological changes and cellular level changes that obviously seem to matter a lot. But what about fMRI, EEG, other kinds of indirect measures, do they count, have they counted as mechanistic evidence?
TIFFANY FARCHIONE: Yeah, so they haven't counted yet. And in part because we just don't have either so far an EEG, fMRI, we see group differences but those aren't the kinds of things that can help predict something for an individual patient.
It just goes back to the whole point about understanding pathophysiology and being able to, you know, not just describe that this drug works on this receptor but also working on this receptor has that relationship downstream to X, Y, and Z effects. And in a clinically meaningful way.
I think ultimately a lot of the things we do in terms of our biomarker qualification program and things like that, understanding not just that a drug has some action or interacts with some sort of biology but in what way and what kind of information does that give you that can help inform the trial or help inform, you know, your assessment of drug effect. That's also important. We're a long way off from being able to put things like that into a drug label I would say.
SARAH “HOLLY” LISANBY: I certainly agree with Dr. Farchione's comments.
And I would like to talk for a moment about devices. And there might be different -- there are different regulations and different considerations in drug design versus device trial design. And we are already at a stage in the field of devices where individual physiology is on the label. And that is the case with the Saint technology where individual resting state functional connectivity MRI is used to target on each patient basis where to put the TMS coil.
And I would say that we -- the jury is still out about, you know, studies that unpack Saint to show where that individualized targeting is essential or whether it's the accelerated intermittent data burst and the ten treatments a day and so on.
Regardless, it is on the label. It's in the instructions for how to use the product. And so I think that that might be a sign of where things may be going in the future. And when we think about the way focal brain stimulation is administered, whether it's non-invasive or surgically implanted, we're targeting circuits in the brain. And being able to measure the impact of that targeting stimulation on the functioning of that circuit, EEG or fMRI might be the right readout and it might give some evidence.
I think even still, though, those measures which may be useful in identifying treatments and optimizing their dosing, ultimately I understand from my FDA colleagues that we'll still need to demonstrate that intervention, whatever it is, improves the quality of life and the clinical aspect for those patients.
But it may be an important part of getting the treatments to that phase where they could be reviewed by FDA.
CAROLYN RODRIGUEZ: Thank you so much. That's a good point. Anyone else to contribute to that? I don't see any other hands raised.
Maybe I'll pass it to Dr. Talkovsky and see if there are any other questions that you see on the Q&A that we could continue to ask the panel.
ALEXANDER TALKOVSKY: Yeah, there was one that jumped out to me a bit earlier. There was a bit of a discussion about warmth and competence as well as a perceived tradeoff between the two. And also some ideas about manipulating them as experimental variables that I thought was interesting. I saw, Dr. Rief, you had jumped into that discussion, too.
I thought that was an important enough topic that would be worth spending a little bit more time here in the group discussion making sure that everybody sees it. So I'll throw it back to you, Dr. Rief.
If you could maybe even elaborate on the answer you gave in there about warmth and competence and those as experimental variables, too.
WINFRIED RIEF: The major point I want to make is that we have to control these variables. If we don't control them, we risk they are different between the two or three arms in our trials. Then we cannot interpret the results. That means we have to assess it and we have to make sure that they are comparable between the different treatments. But this is something I can really recommend, I think it makes a lot of sense. There are other points I'm not sure what to recommend. Some people suggest limit, minimize warmth and competence to minimize potential placebo effects. This is the point where the tradeoff comes into the game. If we minimize warmth and competence, people are not motivated to participate and they might discontinue treatments and they are not willing to cope with side effects.
But if we maximize warmth and competence, we risk that placebo effect is bolstering everything. So at this level, at this stage I would say let's try to keep it in an average level. But really assess it and make sure that it's comparable between the different treatment arms.
ALEXANDER TALKOVSKY: Dr. Atlas, I see your hand up.
LAUREN ATLAS: Yeah. I love this question because I think it depends what the goal is. So if the goal is to reduce placebo to find the best benefit of the drug, then yes, you know, in clinical trials when people never see the same rater, for instance, that reduces the likelihood of building relationship. And there's all these different kinds of features that if you really want to minimize placebo then we can use these things in that way.
On the other hand, if the goal is to have the best patient outcomes, then I think we want to do the exact opposite and essentially identify exactly how these features improve patient's wellbeing and heighten them. And so I think really that is part of why I think talking about placebo is so fascinating because it both tells us how to improve patient outcomes and then also reduce them in the context of trials. So I think it really depends kind of what context you're talking about.
ALEXANDER TALKOVSKY: Dr. Rief.
WINFRIED RIEF: Yeah, may I just add a point. Because I missed it and Lauren reminded me to that point.
Most of us assume that we have to reduce the placebo effects to maximize the difference between placebo and drug effects. And this is an assumption. This is not something that we really know. That means -- and we have studied -- for instance, have seen studies in antidepressants and SSRI. We know studies for analgesics. If you reduce the placebo mechanisms to minimum then you are not able to show a difference to the drug afterward because the drug effects are reduced.
In other words, a good drug needs some minimum of placebo mechanisms to show its full action. Therefore, the assumption that minimizing placebo mechanisms to increase the difference between placebo and drugs is an assumption that we have to be concerned about that. And maybe for some drugs it's much better to have kind of average amount of placebo mechanisms.
ALEXANDER TALKOVSKY: Dr. Wager, let's go to you. Then I think we have another question that we want to tackle in the chat after you wrap up.
TOR WAGER: Yep, that sounds good. I see it, too. But just to weigh in on this. Because I think this is one of the most important issues to me. And I think Winfried also just wrote a review about this. And there have been a couple of others. Which is that there is always this tendency to want to screen out placebo responders. It doesn't seem to work very well most of the time in clinical trials.
And if you have a synergistic interaction over additive interaction between an active drug element and a placebo factor motivation or expectation, then screening out -- that is when screening out placebo responders also screens out the drug responders.
And so I think there is this opportunity to test this more, to test, you know, jointly the effects of active treatments whether it's neuromodulation or drugs or something else. And factors like expectations or perceived warmth and competence of the care provider.
So I guess I'm wondering if in the neurostimulation world are there many studies like that or any studies like that because they seem to be very separate worlds, right? You either study the device or you study the psychosocial aspects.
SARAH “HOLLY”LISANBY: Well, I can and maybe others can as well. It's a good point. Lauren, your talk was really beautiful. And my take-home point from that is in a device trial even if we're not studying the effect of the device operator, the effect is occurring in the trial.
And so measuring these aspects of the whole context of care I think can help us sort that out. And in order to do that, I think it could be helpful for investigators who are designing device trials to partner with investigators who have that expertise. Also in terms of the expertise, I was listening very carefully to the talks about psychosocial interventions and maybe the ancillary effects of the procedure is like a psychosocial intervention that we might benefit from having mixed methods approaches that pull from both fields to really better understand what we're doing.
And then there are also trials that use drugs and devices together. So being able to have cross-pollination across the fields I think would be very useful both with respect to our selection of measures to test the integrity of the blind as well as looking at expectancy and even measuring anything about the provider which is usually not done I would just say for device studies. We're usually not even reporting anything about the provider or the perceptions of the subject about the context of their care.
CAROLYN RODRIGUEZ: I wanted to also jump in, in terms of, you know, just in terms of topics. For psychedelic assisted therapy, Harriet DeWitt has a very good question here in terms of commenting about special considerations and testing of placebos. This is something that has come up a lot. And Boris Heifets, among others, has, you know, really gotten us to think about different kinds of designs to disguise the effects of ketamine, for example, with general anesthesia. There's other designs. But questions around the space.
So how important is it when you have a very active placebo that can have empathogenic effects or psychedelic effects in terms of the placebo effect?
TIFFANY FARCHIONE: Yeah, I figure I should probably jump in on this one first.
So, you know, I will say that when it comes to the psychedelics whether it's a classic psychedelic like psilocybin or if it's the empathogen or tactogen types like MDMA, blinding is practically impossible. Folks know if they are on active drug or a placebo. And that makes it really challenging to have an adequate and well-controlled study, right?
On the one hand, we still need to have placebo-controlled studies so that we can get a fairly -- as accurate as you can get assessment of safety of the drug. On the other hand, we've really been struggling trying to figure out what is the best design. Trying to add some kind of an active comparator, you know, choosing something that might mimic some aspect of the psychedelic effect without actually having a treatment effect of any kind is next to impossible. People still know. You know, you've talked about anything from niacin or benzos, a little bit of this, a little bit of that. They know. They just know.
So the best that we've come up with so far is asking for at least one placebo-controlled study so we can get a clear idea of safety. And we've suggested trying to use complementary designs. For instance, you know, it is still possible to have a dose response study serve as an adequate and well-controlled study. Then there is no placebo there. If you can see that a lower dose, mid dose, high dose, if there is a linear increase in treatment effect in that kind of a study, that is helpful to us. If we have -- one of the other things we ask for is to have some assessment of, you know, like an unblinding questionnaire. Do you think you got the active drug? Yes or no. Do you think you got placebo?
And then one of the things we're starting to ask for now in addition to that is not just assessment at the end of whether folks thought they were on active drug or not, not just from the patient but also from the raters trying to see. Because a lot of times the raters can figure out what the person was on, too, so that could introduce some bias.
Now we're starting to think about asking for like a pre-dose expectancy questionnaire of some kind. And so even if we can't necessarily control for the unblinding issues and the expectancy and everything, at least we can try to -- we can have more data to assess the impact on the study and use those as maybe, you know, covariants in the analyses. But yeah, we don't have the right answer yet. We are learning as we go and we are learning very rapidly.
CAROLYN RODRIGUEZ: That may be a plug for NIMH to do like another -- this placebo panel is amazing. We could keep going. I see we have nine minutes left. I'm going to pass it back to Dr. Talkovsky.
And but I know Dr. Lisanby and Dr. Wager have their hands up so I'll pass it back to Alex.
ALEXANDER TALKOVSKY: Thank you. Because we're short on time, with apologies, Dr. Lisanby and Dr. Wager, there is a question I want to address from the Q&A box that I saw a couple of our panelists already addressed in text but seems worth bringing up here as a group.
Are we confident that the placebo effect and specific affect are additive and not interactive?
LAUREN ATLAS: So I'll just -- can I -- oh, sorry.
CAROLYN RODRIGUEZ: Dr. Atlas, yes, that was quick. You won the buzzer.
ALEXANDER TALKOVSKY: Yes, start us off.
LAUREN ATLAS: I had already responded and was putting something in the chat kind of addressing the dose in the same context.
So basically one approach for testing additivity is to use the balanced placebo design so people receive drug or control and that is crossed with instructions about drug administration. So basically people receive the drug under open administration and they also receive placebo. And they receive the drug when they believe they are not getting treatment leading to hidden administration.
And this has been tested with nicotine effects on -- so nicotine, caffeine. We've done it in the context of remifentanil. There has been a couple other trials of different analgesics. It was really developed in the context of studies of alcohol.
We found, for instance, that depending on the endpoint, we have different conclusions about additivity. So when it came to pain, we found additive effects on pain. But we found pure drug effects on neurologic pain signature responses during remifentanil regardless of whether people knew they were receiving the drug or not. We found interactions when we looked at effects on intention.
And other groups, Christian’s group, has found interactions when they did the same exact trial but used lidocaine. And then furthermore, this is what I think we were just talking about in the context of doses. If people have unblinding at higher doses then there is going to be less of an effect of the context surrounding it. So expectations could grow with higher drug effects.
So I think that the question of additivity or interactions really may depend on the dose, the specific drug, and the specific endpoint. I don't think we can really conclude that.
And so even though doing balanced placebo designs do require a level of deception, I think there is really an urgent need to kind of understand how expectations combine with drugs to influence outcomes.
So yeah, I'm really glad somebody asked that question.
CAROLYN RODRIGUEZ: Thank you, Dr. Atlas. I just want to acknowledge Dr. Cristina Cusin who is the other cochair for the panel. She's on, and I want to be mindful of the time and make sure that she and Dr. Wager have the final words or thoughts or if you want to give the panelist the thoughts.
But we wanted to just pass it back to you so you have plenty of time to say any of the things that you wanted to say to wrap things up.
CRISTINA CUSIN: I will leave to Tor if he has any concluding remarks. My job will be to summarize the wonderful presentation from today and do a brief overview of the meeting tomorrow. It was amazing.
TOR WAGER: Since we have a few minutes left, I would like to go back to what Holly was going to say. We have about five minutes. I'd love to use that time to continue that conversation.
SARAH “HOLLY”LISANBY: I'm assuming that you're referring to the psychedelic question. I agree there is no perfect answer to that and it's very complicated. And there are different views on how to address it.
One of my concerns is therapist unblinding and the potential impact of therapist unblinding on the therapy that is being administered. And because as we've heard, it's very likely that the patient receiving a psychedelic intervention may be unblinded. So might the therapist because they know what a patient going through psychedelic assisted therapy typically experiences.
And one thought I have about that could be to measure the therapy, record it, quantify adherence to the manual. At least document what is going on in the therapy interaction. That would give you some data that might help you interpret and better understand whether therapist unblinding is impacting the psychosocial aspects of the intervention because we do -- we've heard from the field that the setting and aspects and context of the use of the psychedelic are an important part. So let's measure that, too.
TOR WAGER: It's really interesting. I want to note there is another -- Boris Heifets has put in the chat there is something that is a different take.
There might be more things to discuss about whether it's possible to blind these things in some ways and some diversity of opinions there. But you can see the chat comment and we can think about that.
I have one other question about that which is that to me I understand the unblinding problem and that seems to be something we're all really concerned about. What about what you call a sensitivity analysis type of design which is if you can independently manipulate expectations or context and maybe some of these other kinds of drug manipulations that induce another kind of experience, right, that is not the target drug, then you can see whether the outcomes are sensitive to those things or not.
So for some outcomes, they might -- it might not matter what you think or feel or whether you had a, you know, crazy experience or not. And if it doesn't, then that is ignorable, right? So you can manipulate that independently. You don't have to blind it out of your, you know, main manipulation. Or it might turn out to be that yes, that outcome is very sensitive to those kinds of manipulations. So I was wondering what you think about this kind of design.
TIFFANY FARCHIONE: I'm not quite sure that I followed that entirely.
TOR WAGER: Yeah, it's really like so you have one that is the psychedelic drug and you don't unblind it. But then you do an independent manipulation to try to manipulate the non-specific factors. If it's, you know, having a, you know, sort of unique experience or having a -- yeah, or just treatment expectations.
TIFFANY FARCHIONE: I guess that's the piece I'm not quite understanding because I'm not sure what you would be manipulating and how you would accomplish that.
TOR WAGER: In the simplest way, the expectation piece is simpler because you can induce expectations in other ways as well, right? By, you know, giving people suggestions that it's going to really impact them. Or, for example, a design that we've used is to say okay, everyone is -- you know, if you get this drug it's going to make you, I don't know, you know, it's going to give you these sort of strange experiences. But if it gives you these experiences, that means it's not working for you, that's bad. Another group you say this is a sign that it's working.
So you take the subjective symptoms and give people different instructions that those are going to be either helpful or harmful and see if that matters.
TIFFANY FARCHIONE: Yeah, I mean I think if you are giving different people different instructions now you are introducing a different source of potential variability so that kind of makes me a little bit nervous.
I guess what I would say is that if somebody had, you know, some sort of creative problem solving approach to dealing with this, I'd love to hear about it. I would love to see a proposal and a protocol. I would say it's probably best to do in an exploratory proof of concept way first before trying to implement a bunch of fancy bells and whistles in a pivotal study that would try to support the actual approval of a product.
But again, because we're learning as we go, we do tend to be pretty open to different design ideas here and different strategies. You know, as long as people are being monitored appropriately because that piece we don't really budge on.
CAROLYN RODRIGUEZ: I see we're at time. Maybe give Dr. Lisanby the last word. Maybe just some food for thought is that maybe it would be nice to have a toolkit to help clinical trialists have some considerations about how to minimize placebo effects would be something nice. Wish list.
SARAH “HOLLY” LISANBY: Yeah, and I just wanted to add to that last question that this is part of why we're sponsoring this workshop. We want to hear from you what are the gaps in the field, what research needs to be done.
Because we are interested in developing safe and effective interventions, be they psychosocial, drug, device or some combination.
And in the research studies that we support use placebos or other forms of control. We're interested in hearing from you where the research gaps are. What sort of manipulations like, Tor, you were talking about, manipulating expectation, to figure out how to do that. All of that is really interesting research topics. Whether that is the design of a pivotal trial or not, doesn't necessarily need to be that.
We're interested in mapping that gap space so we can figure out how to be most helpful to the field.
TOR WAGER: That's a great last word. We still have tomorrow to solve it all. Hope you all join us tomorrow. Looking forward to it. Thank you.
(Adjourned)
Information
- Author Services
Initiatives
You are accessing a machine-readable page. In order to be human-readable, please install an RSS reader.
All articles published by MDPI are made immediately available worldwide under an open access license. No special permission is required to reuse all or part of the article published by MDPI, including figures and tables. For articles published under an open access Creative Common CC BY license, any part of the article may be reused without permission provided that the original article is clearly cited. For more information, please refer to https://www.mdpi.com/openaccess .
Feature papers represent the most advanced research with significant potential for high impact in the field. A Feature Paper should be a substantial original Article that involves several techniques or approaches, provides an outlook for future research directions and describes possible research applications.
Feature papers are submitted upon individual invitation or recommendation by the scientific editors and must receive positive feedback from the reviewers.
Editor’s Choice articles are based on recommendations by the scientific editors of MDPI journals from around the world. Editors select a small number of articles recently published in the journal that they believe will be particularly interesting to readers, or important in the respective research area. The aim is to provide a snapshot of some of the most exciting work published in the various research areas of the journal.
Original Submission Date Received: .
- Active Journals
- Find a Journal
- Proceedings Series
- For Authors
- For Reviewers
- For Editors
- For Librarians
- For Publishers
- For Societies
- For Conference Organizers
- Open Access Policy
- Institutional Open Access Program
- Special Issues Guidelines
- Editorial Process
- Research and Publication Ethics
- Article Processing Charges
- Testimonials
- Preprints.org
- SciProfiles
- Encyclopedia
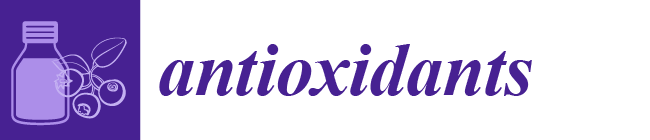
Article Menu
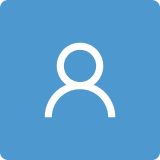
- Subscribe SciFeed
- Recommended Articles
- Google Scholar
- on Google Scholar
- Table of Contents
Find support for a specific problem in the support section of our website.
Please let us know what you think of our products and services.
Visit our dedicated information section to learn more about MDPI.
JSmol Viewer
Downregulation of iron–sulfur cluster biogenesis may contribute to hyperglycemia-mediated diabetic peripheral neuropathy in murine models.
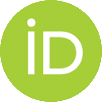
1. Introduction
2. materials and methods, 2.1. animals, 2.2. glucose and insulin tolerance tests, 2.3. hot plate test, 2.4. rotarod test, 2.5. beam-walk test, 2.6. electron microscopy, 2.7. histological assays, 2.8. ferrozine iron assays, 2.9. labile iron pool (lip) measurements, 2.10. the enzymatic activities of aconitase, complexes i and ii, and mitochondrial membrane potential (mmp) levels, 2.11. ros detection assays, 2.12. western blot assays, 2.13. quantitative real-time pcr, 2.14. statistics analysis, 3.1. high glucose downregulates fe-s-cluster biogenesis and fe-s-containing enzymatic activities in rsc96 cells, 3.2. high-glucose treatment increases the cellular labile iron pool and promotes ros production in rsc96 cells, 3.3. pioglitazone treatment increases the expression of genes involved in the biosynthesis of fe-s clusters and recovers iron homeostasis and redox balance in rsc96 cells, 3.4. rescue of fe-s cluster biosynthesis by pioglitazone treatment correlates well with the increase in the enzymatic activities of fe-s protein and with the improvement of iron homeostasis and inflammation in sciatic nerve, 4. discussion, 5. conclusions, supplementary materials, author contributions, institutional review board statement, informed consent statement, data availability statement, acknowledgments, conflicts of interest.
- Elafros, M.A.; Andersen, H.; Bennett, D.L.; Savelieff, M.G.; Viswanathan, V.; Callaghan, B.C.; Feldman, E.L. Towards prevention of diabetic peripheral neuropathy: Clinical presentation, pathogenesis, and new treatments. Lancet Neurol. 2022 , 21 , 922–936. [ Google Scholar ] [ CrossRef ] [ PubMed ]
- Selvarajah, D.; Kar, D.; Khunti, K.; Davies, M.J.; Scott, A.R.; Walker, J.; Tesfaye, S. Diabetic peripheral neuropathy: Advances in diagnosis and strategies for screening and early intervention. Lancet Diabetes Endocrinol. 2019 , 7 , 938–948. [ Google Scholar ] [ CrossRef ] [ PubMed ]
- Majd, H.; Amin, S.; Ghazizadeh, Z.; Cesiulis, A.; Arroyo, E.; Lankford, K.; Majd, A.; Farahvashi, S.; Chemel, A.K.; Okoye, M.; et al. Deriving Schwann cells from hPSCs enables disease modeling and drug discovery for diabetic peripheral neuropathy. Cell Stem Cell 2023 , 30 , 632–647.e10. [ Google Scholar ] [ CrossRef ]
- Li, K. Iron Pathophysiology in Friedreich’s Ataxia. Adv. Exp. Med. Biol. 2019 , 1173 , 125–143. [ Google Scholar ] [ CrossRef ] [ PubMed ]
- Lill, R.; Freibert, S.A. Mechanisms of Mitochondrial Iron-Sulfur Protein Biogenesis. Annu. Rev. Biochem. 2020 , 89 , 471–499. [ Google Scholar ] [ CrossRef ]
- Mochel, F.; Knight, M.A.; Tong, W.H.; Hernandez, D.; Ayyad, K.; Taivassalo, T.; Andersen, P.M.; Singleton, A.; Rouault, T.A.; Fischbeck, K.H.; et al. Splice mutation in the iron-sulfur cluster scaffold protein ISCU causes myopathy with exercise intolerance. Am. J. Hum. Genet. 2008 , 82 , 652–660. [ Google Scholar ] [ CrossRef ]
- Obis, È.; Irazusta, V.; Sanchís, D.; Ros, J.; Tamarit, J. Frataxin deficiency in neonatal rat ventricular myocytes targets mitochondria and lipid metabolism. Free Radic. Biol. Med. 2014 , 73 , 21–33. [ Google Scholar ] [ CrossRef ]
- Wong, A.; Yang, J.; Cavadini, P.; Gellera, C.; Lonnerdal, B.; Taroni, F.; Cortopassi, G. The Friedreich’s ataxia mutation confers cellular sensitivity to oxidant stress which is rescued by chelators of iron and calcium and inhibitors of apoptosis. Hum. Mol. Genet. 1999 , 8 , 425–430. [ Google Scholar ] [ CrossRef ]
- Chantrel-Groussard, K.; Geromel, V.; Puccio, H.; Koenig, M.; Munnich, A.; Rötig, A.; Rustin, P. Disabled early recruitment of antioxidant defenses in Friedreich’s ataxia. Hum. Mol. Genet. 2001 , 10 , 2061–2067. [ Google Scholar ] [ CrossRef ]
- Koeppen, A.H.; Mazurkiewicz, J.E. Friedreich ataxia: Neuropathology revised. J. Neuropathol. Exp. Neurol. 2013 , 72 , 78–90. [ Google Scholar ] [ CrossRef ]
- Koeppen, A.H.; Morral, J.A.; Davis, A.N.; Qian, J.; Petrocine, S.V.; Knutson, M.D.; Gibson, W.M.; Cusack, M.J.; Li, D. The dorsal root ganglion in Friedreich’s ataxia. Acta Neuropathol. 2009 , 118 , 763–776. [ Google Scholar ] [ CrossRef ] [ PubMed ]
- Rosenblatt, S.; Miskin, B.; Glazer, N.B.; Prince, M.J.; Robertson, K.E. The impact of pioglitazone on glycemic control and atherogenic dyslipidemia in patients with type 2 diabetes mellitus. Coron. Artery Dis. 2001 , 12 , 413–423. [ Google Scholar ] [ CrossRef ] [ PubMed ]
- Poupon-Bejuit, L.; Rocha-Ferreira, E.; Thornton, C.; Hagberg, H.; Rahim, A.A. Neuroprotective Effects of Diabetes Drugs for the Treatment of Neonatal Hypoxia-Ischemia Encephalopathy. Front. Cell. Neurosci. 2020 , 14 , 112. [ Google Scholar ] [ CrossRef ]
- Kuate Defo, A.; Bakula, V.; Pisaturo, A.; Labos, C.; Wing, S.S.; Daskalopoulou, S.S. Diabetes, antidiabetic medications and risk of dementia: A systematic umbrella review and meta-analysis. Diabetes Obes. Metab. 2024 , 26 , 441–462. [ Google Scholar ] [ CrossRef ] [ PubMed ]
- Fadini, G.P.; Longato, E.; Morieri, M.L.; Del Prato, S.; Avogaro, A.; Solini, A. Long-term benefits of dapagliflozin on renal outcomes of type 2 diabetes under routine care: A comparative effectiveness study on propensity score matched cohorts at low renal risk. Lancet Reg. Health Eur. 2024 , 38 , 100847. [ Google Scholar ] [ CrossRef ]
- Sun, Y.G.; Zhao, Z.Q.; Meng, X.L.; Yin, J.; Liu, X.Y.; Chen, Z.F. Cellular basis of itch sensation. Science 2009 , 325 , 1531–1534. [ Google Scholar ] [ CrossRef ]
- Liu, Y.; Cai, J.; Shen, J.; Dong, W.; Xu, L.; Fang, M.; Lin, Y.; Liu, J.; Ding, Y.; Qiao, T.; et al. SS-31 efficacy in a mouse model of Friedreich ataxia by upregulation of frataxin expression. Hum. Mol. Genet. 2021 , 31 , 176–188. [ Google Scholar ] [ CrossRef ]
- Xu, L.; Sun, Z.; Xing, Z.; Liu, Y.; Zhao, H.; Tang, Z.; Luo, Y.; Hao, S.; Li, K. Cur@SF NPs alleviate Friedreich’s ataxia in a mouse model through synergistic iron chelation and antioxidation. J. Nanobiotechnol. 2022 , 20 , 118. [ Google Scholar ] [ CrossRef ] [ PubMed ]
- Li, H.; Zhao, H.; Hao, S.; Shang, L.; Wu, J.; Song, C.; Meyron-Holtz, E.G.; Qiao, T.; Li, K. Iron regulatory protein deficiency compromises mitochondrial function in murine embryonic fibroblasts. Sci. Rep. 2018 , 8 , 5118. [ Google Scholar ] [ CrossRef ]
- Li, H.; Liu, Y.; Shang, L.; Cai, J.; Wu, J.; Zhang, W.; Pu, X.; Dong, W.; Qiao, T.; Li, K. Iron regulatory protein 2 modulates the switch from aerobic glycolysis to oxidative phosphorylation in mouse embryonic fibroblasts. Proc. Natl. Acad. Sci. USA 2019 , 116 , 9871–9876. [ Google Scholar ] [ CrossRef ]
- Yin, Y.; Qu, H.; Yang, Q.; Fang, Z.; Gao, R. Astragaloside IV alleviates Schwann cell injury in diabetic peripheral neuropathy by regulating microRNA-155-mediated autophagy. Phytomedicine 2021 , 92 , 153749. [ Google Scholar ] [ CrossRef ]
- Lin, Q.; Li, K.; Chen, Y.; Xie, J.; Wu, C.; Cui, C.; Deng, B. Oxidative Stress in Diabetic Peripheral Neuropathy: Pathway and Mechanism-Based Treatment. Mol. Neurobiol. 2023 , 60 , 4574–4594. [ Google Scholar ] [ CrossRef ]
- Rodríguez-Pascau, L.; Britti, E.; Calap-Quintana, P.; Dong, Y.N.; Vergara, C.; Delaspre, F.; Medina-Carbonero, M.; Tamarit, J.; Pallardó, F.V.; Gonzalez-Cabo, P.; et al. PPAR gamma agonist leriglitazone improves frataxin-loss impairments in cellular and animal models of Friedreich Ataxia. Neurobiol. Dis. 2021 , 148 , 105162. [ Google Scholar ] [ CrossRef ]
- Marmolino, D.; Acquaviva, F.; Pinelli, M.; Monticelli, A.; Castaldo, I.; Filla, A.; Cocozza, S. PPAR-gamma agonist Azelaoyl PAF increases frataxin protein and mRNA expression: New implications for the Friedreich’s ataxia therapy. Cerebellum 2009 , 8 , 98–103. [ Google Scholar ] [ CrossRef ]
- Kawasaki, F.; Matsuda, M.; Kanda, Y.; Inoue, H.; Kaku, K. Structural and functional analysis of pancreatic islets preserved by pioglitazone in db/db mice. Am. J. Physiol. Endocrinol. Metab. 2005 , 288 , E510–E518. [ Google Scholar ] [ CrossRef ]
- Hinder, L.M.; Park, M.; Rumora, A.E.; Hur, J.; Eichinger, F.; Pennathur, S.; Kretzler, M.; Brosius, F.C., 3rd; Feldman, E.L. Comparative RNA-Seq transcriptome analyses reveal distinct metabolic pathways in diabetic nerve and kidney disease. J. Cell. Mol. Med. 2017 , 21 , 2140–2152. [ Google Scholar ] [ CrossRef ]
- Mert, T.; Sahin, E.; Yaman, S.; Sahin, M. Effects of immune cell-targeted treatments result from the suppression of neuronal oxidative stress and inflammation in experimental diabetic rats. Naunyn Schmiedebergs Arch. Pharmacol. 2020 , 393 , 1293–1302. [ Google Scholar ] [ CrossRef ]
- Cashman, C.R.; Höke, A. Mechanisms of distal axonal degeneration in peripheral neuropathies. Neurosci. Lett. 2015 , 596 , 33–50. [ Google Scholar ] [ CrossRef ]
- Lennertz, R.C.; Medler, K.A.; Bain, J.L.; Wright, D.E.; Stucky, C.L. Impaired sensory nerve function and axon morphology in mice with diabetic neuropathy. J. Neurophysiol. 2011 , 106 , 905–914. [ Google Scholar ] [ CrossRef ]
- Xu, X.F.; Zhang, D.D.; Liao, J.C.; Xiao, L.; Wang, Q.; Qiu, W. Galanin and its receptor system promote the repair of injured sciatic nerves in diabetic rats. Neural Regen. Res. 2016 , 11 , 1517–1526. [ Google Scholar ] [ CrossRef ]
- Guo, D.; Lu, X.; Xu, X.; Gou, H.; Wang, Z.; Cao, Y.; Luo, X. Therapeutic Effect of Vinorine on Sciatic Nerve Injured Rat. Neurochem. Res. 2018 , 43 , 375–386. [ Google Scholar ] [ CrossRef ] [ PubMed ]
- Rolim, L.C.; da Silva, E.M.; Flumignan, R.L.; Abreu, M.M.; Dib, S.A. Acetyl-L-carnitine for the treatment of diabetic peripheral neuropathy. Cochrane Database Syst. Rev. 2019 , 6 , Cd011265. [ Google Scholar ] [ CrossRef ]
- Cnop, M.; Mulder, H.; Igoillo-Esteve, M. Diabetes in Friedreich ataxia. J. Neurochem. 2013 , 126 (Suppl. S1), 94–102. [ Google Scholar ] [ CrossRef ]
- Turchi, R.; Tortolici, F.; Guidobaldi, G.; Iacovelli, F.; Falconi, M.; Rufini, S.; Faraonio, R.; Casagrande, V.; Federici, M.; De Angelis, L.; et al. Frataxin deficiency induces lipid accumulation and affects thermogenesis in brown adipose tissue. Cell Death Dis. 2020 , 11 , 51. [ Google Scholar ] [ CrossRef ]
- DeFronzo, R.A.; Inzucchi, S.; Abdul-Ghani, M.; Nissen, S.E. Pioglitazone: The forgotten, cost-effective cardioprotective drug for type 2 diabetes. Diabetes Vasc. Dis. Res. 2019 , 16 , 133–143. [ Google Scholar ] [ CrossRef ]
Click here to enlarge figure
The statements, opinions and data contained in all publications are solely those of the individual author(s) and contributor(s) and not of MDPI and/or the editor(s). MDPI and/or the editor(s) disclaim responsibility for any injury to people or property resulting from any ideas, methods, instructions or products referred to in the content. |
Share and Cite
Wu, L.; Huang, F.; Sun, Z.; Zhang, J.; Xia, S.; Zhao, H.; Liu, Y.; Yang, L.; Ding, Y.; Bian, D.; et al. Downregulation of Iron–Sulfur Cluster Biogenesis May Contribute to Hyperglycemia-Mediated Diabetic Peripheral Neuropathy in Murine Models. Antioxidants 2024 , 13 , 1036. https://doi.org/10.3390/antiox13091036
Wu L, Huang F, Sun Z, Zhang J, Xia S, Zhao H, Liu Y, Yang L, Ding Y, Bian D, et al. Downregulation of Iron–Sulfur Cluster Biogenesis May Contribute to Hyperglycemia-Mediated Diabetic Peripheral Neuropathy in Murine Models. Antioxidants . 2024; 13(9):1036. https://doi.org/10.3390/antiox13091036
Wu, Lin, Fei Huang, Zichen Sun, Jinghua Zhang, Siyu Xia, Hongting Zhao, Yutong Liu, Lu Yang, Yibing Ding, Dezhi Bian, and et al. 2024. "Downregulation of Iron–Sulfur Cluster Biogenesis May Contribute to Hyperglycemia-Mediated Diabetic Peripheral Neuropathy in Murine Models" Antioxidants 13, no. 9: 1036. https://doi.org/10.3390/antiox13091036
Article Metrics
Article access statistics, supplementary material.
ZIP-Document (ZIP, 149 KiB)
Further Information
Mdpi initiatives, follow mdpi.
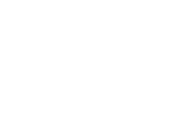
Subscribe to receive issue release notifications and newsletters from MDPI journals

An official website of the United States government
The .gov means it’s official. Federal government websites often end in .gov or .mil. Before sharing sensitive information, make sure you’re on a federal government site.
The site is secure. The https:// ensures that you are connecting to the official website and that any information you provide is encrypted and transmitted securely.
- Publications
- Account settings
- My Bibliography
- Collections
- Citation manager
Save citation to file
Email citation, add to collections.
- Create a new collection
- Add to an existing collection
Add to My Bibliography
Your saved search, create a file for external citation management software, your rss feed.
- Search in PubMed
- Search in NLM Catalog
- Add to Search
Diosmetin attenuates fibromyalgia-like symptoms in a reserpine-induced model in mice
Affiliations.
- 1 Neurotoxicity and Psychopharmacology Laboratory-Pain Research Group, Centre of Natural and Exact Sciences, Federal University of Santa Maria, Santa Maria, RS, Brazil.
- 2 Graduate Program in Biological Sciences: Toxicological Biochemistry, Centre of Natural and Exact Sciences, Federal University of Santa Maria, Santa Maria, RS, Brazil.
- 3 Department of Microbiology and Parasitology, Federal University of Santa Maria, Santa Maria, RS, Brazil.
- 4 Department of Biochemistry and Molecular Biology, Centre of Natural and Exact Sciences, Federal University of Santa Maria, Santa Maria, RS, Camobi, 97105-900, Brazil.
- 5 Neurotoxicity and Psychopharmacology Laboratory-Pain Research Group, Centre of Natural and Exact Sciences, Federal University of Santa Maria, Santa Maria, RS, Brazil. [email protected].
- 6 Graduate Program in Biological Sciences: Toxicological Biochemistry, Centre of Natural and Exact Sciences, Federal University of Santa Maria, Santa Maria, RS, Brazil. [email protected].
- 7 Department of Biochemistry and Molecular Biology, Centre of Natural and Exact Sciences, Federal University of Santa Maria, Santa Maria, RS, Camobi, 97105-900, Brazil. [email protected].
- PMID: 38662182
- DOI: 10.1007/s10787-024-01473-4
Fibromyalgia is a potentially disabling idiopathic disease characterized by widespread chronic pain associated with comorbidities such as fatigue, anxiety, and depression. Current therapeutic approaches present adverse effects that limit adherence to therapy. Diosmetin, an aglycone of the flavonoid glycoside diosmin found in citrus fruits and the leaves of Olea europaea L., has antinociceptive, anti-inflammatory, and antioxidant properties. Here, we investigated the effect of diosmetin on nociceptive behaviors and comorbidities in an experimental fibromyalgia model induced by reserpine in mice. To induce the experimental fibromyalgia model, a protocol of subcutaneous injections of reserpine (1 mg/kg) was used once a day for three consecutive days in adult male Swiss mice. Mice received oral diosmetin on the fourth day after the first reserpine injection. Nociceptive (mechanical allodynia, muscle strength, and thermal hyperalgesia) and comorbid (depressive-like and anxiety behavior) parameters were evaluated. Potential adverse effects associated with diosmetin plus reserpine (locomotor alteration, cataleptic behavior, and body weight and temperature changes) were also evaluated. Oral diosmetin (0.015-1.5 mg/kg) reduced the mechanical allodynia, thermal hyperalgesia, and loss of muscle strength induced by reserpine. Diosmetin (0.15 mg/kg) also attenuated depressive-like and anxiety behaviors without causing locomotor alteration, cataleptic behavior, and alteration in weight and body temperature of mice. Overall, diosmetin can be an effective and safe therapeutic alternative to treat fibromyalgia symptoms, such as pain, depression and anxiety.
Keywords: Allodynia; Analgesic; Anxiety; Depression; Natural products.
© 2024. The Author(s), under exclusive licence to Springer Nature Switzerland AG.
PubMed Disclaimer
Similar articles
- Broad-spectrum cannabis oil ameliorates reserpine-induced fibromyalgia model in mice. Ferrarini EG, Paes RS, Baldasso GM, de Assis PM, Gouvêa MC, Cicco P, Raposo NRB, Capasso R, Moreira ELG, Dutra RC. Ferrarini EG, et al. Biomed Pharmacother. 2022 Oct;154:113552. doi: 10.1016/j.biopha.2022.113552. Epub 2022 Aug 18. Biomed Pharmacother. 2022. PMID: 35988425
- Involvement of TRPV1 and the efficacy of α-spinasterol on experimental fibromyalgia symptoms in mice. Fischer SPM, Brusco I, Brum ES, Fialho MFP, Camponogara C, Scussel R, Machado-de-Ávila RA, Trevisan G, Oliveira SM. Fischer SPM, et al. Neurochem Int. 2020 Mar;134:104673. doi: 10.1016/j.neuint.2020.104673. Epub 2020 Jan 8. Neurochem Int. 2020. PMID: 31926196
- Topical diosmetin attenuates nociception and inflammation in a ultraviolet B radiation-induced sunburn model in mice. Favarin A, Becker G, Brum ES, Serafini PT, Marquezin LP, Brusco I, Oliveira SM. Favarin A, et al. Inflammopharmacology. 2024 Aug;32(4):2295-2304. doi: 10.1007/s10787-024-01507-x. Epub 2024 Jun 22. Inflammopharmacology. 2024. PMID: 38907857
- Unveiling the Molecular Mechanism of Diosmetin and its Impact on Multifaceted Cellular Signaling Pathways. Varshney KK, Gupta JK, Srivastava R. Varshney KK, et al. Protein Pept Lett. 2024;31(4):275-289. doi: 10.2174/0109298665294109240323033601. Protein Pept Lett. 2024. PMID: 38629379 Review.
- Diosmetin: A dietary flavone as modulator of signaling pathways in cancer progression. Raza W, Meena A, Luqman S. Raza W, et al. Mol Carcinog. 2024 Sep;63(9):1627-1642. doi: 10.1002/mc.23774. Epub 2024 Jun 18. Mol Carcinog. 2024. PMID: 38888206 Review.
- Adamante G, de Almeida AS, Rigo FK et al (2019) Diosmetin as a novel transient receptor potential vanilloid 1 antagonist with antinociceptive activity in mice. Life Sci 216:215–226. https://doi.org/10.1016/j.lfs.2018.11.029 - DOI - PubMed
- Arnold LM, Gebke KB, Choy EHS (2016) Fibromyalgia: Management strategies for primary care providers. Int J Clin Pract 70:99–112. https://doi.org/10.1111/IJCP.12757 - DOI - PubMed
- Atwal N, Casey SL, Mitchell VA, Vaughan CW (2019) THC and gabapentin interactions in a mouse neuropathic pain model. Neuropharmacology 144:115–121. https://doi.org/10.1016/j.neuropharm.2018.10.006 - DOI - PubMed
- Brederson J-D, Jarvis FM, Honore PS, Surowy C (2011) Fibromyalgia: mechanisms, current treatment and animal models. Curr Pharm Biotechnol 12:1613–1626. https://doi.org/10.2174/138920111798357258 - DOI - PubMed
- Brum ES, Fialho MFP, Fischer SPM et al (2020) Relevance of Mitochondrial Dysfunction in the Reserpine-Induced Experimental Fibromyalgia Model. Mol Neurobiol 57(4202):4217. https://doi.org/10.1007/s12035-020-01996-1 - DOI
- Search in MeSH
Related information
- PubChem Compound (MeSH Keyword)
Grants and funding
- #23038.002125/2021-85/Coordenação de Aperfeiçoamento de Pessoal de Nível Superior
- Grant #0036/2021/Coordenação de Aperfeiçoamento de Pessoal de Nível Superior
- #88882.182170/2018-01/Coordenação de Aperfeiçoamento de Pessoal de Nível Superior
- #21/2551-0001966-2/Fundação de Amparo à Pesquisa do Estado do Rio Grande do Sul
- #304985/2020-1/Conselho Nacional de Desenvolvimento Científico e Tecnológico
- #307690/2021-0/Conselho Nacional de Desenvolvimento Científico e Tecnológico
LinkOut - more resources
- MedlinePlus Health Information
- Citation Manager
NCBI Literature Resources
MeSH PMC Bookshelf Disclaimer
The PubMed wordmark and PubMed logo are registered trademarks of the U.S. Department of Health and Human Services (HHS). Unauthorized use of these marks is strictly prohibited.

IMAGES
VIDEO
COMMENTS
Animal models of pain are designed to mimic distinct clinical diseases to better evaluate underlying mechanisms and potential treatments. ... Levine B, Henry JL, Desevre J, Kroin JS. Alteration of sensory neurons and spinal response to an experimental osteoarthritis pain model. Arthritis Rheum. 2010; 62:2995-3005. [PMC free article] [Google ...
Animal models of pain are crucially important for progress in the field, but the poor translation record of pain research has led to a re-evaluation of the appropriateness of current models. The ...
Fig. 1: Analysis of rodent use in pain studies from 1980 to 2020. The methods and results sections of preclinical reports published in the journal Pain in the years 1980, 1990, 2000, 2010 and 2016 ...
The first characterization of pain in an animal model of pain found that experimental ischemia of the spinal cord caused significant allodynia (Hao et al. 1991). This model is established by intravenously injecting rats with a fluorescein dye then immediately irradiating the exposed T10 vertebrae, destroying vascular innervation to the target ...
The humane use of preclinical animal models plays a critical role both in understanding the basic biology of pain as well as in the development of therapeutic treatments to alleviate pain. Clinically relevant pain is the result of complex processes involving peripheral transduction and transmission as well as central modulation and processing that leads to the final conscious sensation of pain.
Refined analysis of animal models of pain and depression has become mandatory to understand interactions between pain and emotional comorbidities (Kremer et al., 2020). ... In experimental pain models, the response changes into sustained lifting, flinching or even licking behavior. Static mechanical sensitivity can be assessed with a pressure ...
cally self‑limiting, and thus laboratory animal models of pain are widely used (BOX 1). However, pain studies ... both experimental and clinical pain16. Nonetheless, the
However, quantitative assessment of pain in animal models is technically and conceptually challenging, and it is only in recent years that inflammatory arthritis models have begun to be utilized systematically in experimental pain studies using behavioral and neurophysiological approaches to characterize acute and chronic pain stages. This ...
Experimental models of pain advance the understanding of the basic science and physiology of pain and aid the development of therapeutic modalities. Most pain research has been conducted in animals and humans, with some in vitro and ex vivo systems that focus on gene expression and neuronal firing [ 1 ]. Model choice should derive from the ...
This article reviews some of the most widely used or promising new models for chronic pain. Partial spinal ligation, chronic constriction injury, and L5/L6 spinal nerve ligation represent three of the best characterized rodent models of peripheral neuropathy. Recently, several mouse and rat bone cancer pain models have been reported.
In general, readouts from experimental animal models vary in sensitivity to analgesics, and so the most critical task is to understand how this reflects the perception and experience of the individual. Good sensitivity of a drug in an animal model does not guarantee the validity, rather indicates that the model is tuned toward a certain pain ...
To facilitate the study of pain transmission and the characterization of novel analgesic compounds, an array of experimental animal pain models has been developed mainly in rodents. In these preclinical models, nociceptive pain can be measured by both spontaneous and evoked behaviors. Acute pain (seconds to hours) can be more easily measured ...
Nociceptive tests utilize observations of animal behavior after delivering noxious mechanical, heat, or chemical stimuli to a defined body part. In this section, we will address a variety of tests used to study nociception and pain. Two of these tests, the allodynia test, mechanical and cold allodynia, and the Randall-Selitto paw pressure test ...
Behavioral assessments are used to evaluate experimental outcomes. They are categorized into reflexive, non-reflexive, free-choice tests, or non-evoked voluntary behaviors, and relate to assessing the varied sensory and emotional experiences of pain [8; 9]. ... Trang T. Animal models of chronic pain: Advances and challenges for clinical ...
The establishment of an ideal experimental animal model in line with the pathophysiological characteristics of clinical visceral diseases is of great significant and critical to explore the mechanisms of induction, maintenance, and regulation in visceral pain, and to further screen the therapeutic drugs for related visceral diseases.
A number of animal models of SCI exist and are primarily used to determine mechanisms of motor dysfunctions [2 - 4]. Recently, these various SCI animal models have been utilized for pain studies . However, when SCI animal models are used for pain research, special attention should be paid to the concomitant conditions.
Animal models - Mimicking the pain of trigeminal neuralgia. Trigeminal neuralgia (TN) is an episodic facial pain which feels like an electric shock of unilateral origin. This neuropathic disorder is an intensely stressful to bear for patient and impacts the quality of life. Most of the cases of TN arise when the root of fifth cranial nerve, i.e ...
Broadly, experimental assessment of pain in animal models relies on the quantification of pain-evoked or pain-suppressed behaviors. Pain-evoked behaviors may occur at very low rates in the absence of pain, and increase in frequency following putative nociceptive stimuli. Examples include footpad withdrawal, jumping, flinching or licking.
Free. Abstract. In Brief. KEHL, L. J., and C. A. FAIRBANKS. Experimental animal models of muscle pain and analgesia. Exerc. Sport Sci. Rev., Vol. 31, No. 4, pp. 188-194, 2003. Muscle pain is a prevalent clinical problem but can be difficult to treat because relatively little is known about nervous system mechanisms that mediate and modulate it.
To study pain transmission, identify new pain targets and characterise the potential analgesic profile of novel compounds, an array of experimental animal pain models has been developed (mainly in rodents) attempting to replicate the many human pain conditions, including inflammatory, neuropathic, visceral and cancer pain states. The authors ...
Unfortunately, TKA surgery is accompanied by acute postoperative pain that is more severe than arthroplasty performed in other joints . Elucidating the molecular mechanisms specific to post-TKA pain necessitates an animal model that replicates clinical TKA procedures, induces acute postoperative pain, and leads to complete functional recovery .
A second model, such as an abdominal incision (laparotomy), would further strengthen our conclusions. Although topical application of our drug QM385 on the back skin resulted in reduced BH4 and serotonin, we were not able to measure direct pain responses in these animals. Lastly, we also observed that activated mast cells release BH4 in vitro.
The value of human experimental pain models is to link animal and clinical pain studies, providing new possibilities for designing successful clinical trials. Spontaneous pain, the main compliant of the neuropathic patients, but currently there is no human model available that would mimic chronic pain. Therefore, current human pain models ...
Animal models of inflammatory pain have been widely used to study the mechanisms of tissue injury-induced persistent pain. ... (2007) Site-specific, dose-dependent, and sex-related responses to the experimental pain model induced by intradermal injection of capsaicin to the foreheads and forearms of healthy humans, Journal of Orofacial Pain 21 ...
The company announced that a recent preclinical study in rats demonstrated that its oral ketamine analog, Ketamir-2, produced a significant reversal of neuropathic pain, induced by nerve ligation ...
Abstract. Muscle pain is a prevalent clinical problem but can be difficult to treat because relatively little is known about nervous system mechanisms that mediate and modulate it. This review profiles four new animal models of muscle and deep tissue pain currently being used to elucidate mechanisms of muscle pain and analgesia.
The National Institute of Mental Health (NIMH) hosted a virtual workshop on the placebo effect. The purpose of this workshop was to bring together experts in neurobiology, clinical trials, and regulatory science to examine placebo effects in drug, device, and psychosocial interventions for mental health conditions. Topics included interpretability of placebo signals within the context of ...
Background: Diabetic peripheral neuropathy (DPN) is considered one of the most common chronic complications of diabetes. Impairment of mitochondrial function is regarded as one of the causes. Iron-sulfur clusters are essential cofactors for numerous iron-sulfur (Fe-S)-containing proteins/enzymes, including mitochondrial electron transport chain complex I, II, and III and aconitase. Methods ...
Here, we investigated the effect of diosmetin on nociceptive behaviors and comorbidities in an experimental fibromyalgia model induced by reserpine in mice. To induce the experimental fibromyalgia model, a protocol of subcutaneous injections of reserpine (1 mg/kg) was used once a day for three consecutive days in adult male Swiss mice.