6.2 Uniform Circular Motion
Section learning objectives.
By the end of this section, you will be able to do the following:
- Describe centripetal acceleration and relate it to linear acceleration
- Describe centripetal force and relate it to linear force
- Solve problems involving centripetal acceleration and centripetal force

Teacher Support
The learning objectives in this section will help your students master the following standards:
- (C) analyze and describe accelerated motion in two dimensions using equations, including projectile and circular examples.
- (D) calculate the effect of forces on objects, including the law of inertia, the relationship between force and acceleration, and the nature of force pairs between objects.
In addition, the High School Physics Laboratory Manual addresses content in this section in the lab titled: Circular and Rotational Motion, as well as the following standards:
Section Key Terms
centrifugal force | centripetal acceleration | centripetal force | uniform circular motion |
Centripetal Acceleration
[BL] [OL] Review uniform circular motion. Ask students to give examples of circular motion. Review linear acceleration.
In the previous section, we defined circular motion . The simplest case of circular motion is uniform circular motion , where an object travels a circular path at a constant speed . Note that, unlike speed, the linear velocity of an object in circular motion is constantly changing because it is always changing direction. We know from kinematics that acceleration is a change in velocity , either in magnitude or in direction or both. Therefore, an object undergoing uniform circular motion is always accelerating, even though the magnitude of its velocity is constant.
You experience this acceleration yourself every time you ride in a car while it turns a corner. If you hold the steering wheel steady during the turn and move at a constant speed, you are executing uniform circular motion. What you notice is a feeling of sliding (or being flung, depending on the speed) away from the center of the turn. This isn’t an actual force that is acting on you—it only happens because your body wants to continue moving in a straight line (as per Newton’s first law) whereas the car is turning off this straight-line path. Inside the car it appears as if you are forced away from the center of the turn. This fictitious force is known as the centrifugal force . The sharper the curve and the greater your speed, the more noticeable this effect becomes.
[BL] [OL] [AL] Demonstrate circular motion by tying a weight to a string and twirling it around. Ask students what would happen if you suddenly cut the string? In which direction would the object travel? Why? What does this say about the direction of acceleration? Ask students to give examples of when they have come across centripetal acceleration.
Figure 6.7 shows an object moving in a circular path at constant speed. The direction of the instantaneous tangential velocity is shown at two points along the path. Acceleration is in the direction of the change in velocity; in this case it points roughly toward the center of rotation. (The center of rotation is at the center of the circular path). If we imagine Δ s Δ s becoming smaller and smaller, then the acceleration would point exactly toward the center of rotation, but this case is hard to draw. We call the acceleration of an object moving in uniform circular motion the centripetal acceleration a c because centripetal means center seeking .
Consider Figure 6.7 . The figure shows an object moving in a circular path at constant speed and the direction of the instantaneous velocity of two points along the path. Acceleration is in the direction of the change in velocity and points toward the center of rotation. This is strictly true only as Δ s Δ s tends to zero.
Now that we know that the direction of centripetal acceleration is toward the center of rotation, let’s discuss the magnitude of centripetal acceleration. For an object traveling at speed v in a circular path with radius r , the magnitude of centripetal acceleration is
Centripetal acceleration is greater at high speeds and in sharp curves (smaller radius), as you may have noticed when driving a car, because the car actually pushes you toward the center of the turn. But it is a bit surprising that a c is proportional to the speed squared. This means, for example, that the acceleration is four times greater when you take a curve at 100 km/h than at 50 km/h.
We can also express a c in terms of the magnitude of angular velocity . Substituting v = r ω v = r ω into the equation above, we get a c = ( r ω ) 2 r = r ω 2 a c = ( r ω ) 2 r = r ω 2 . Therefore, the magnitude of centripetal acceleration in terms of the magnitude of angular velocity is
Tips For Success
The equation expressed in the form a c = rω 2 is useful for solving problems where you know the angular velocity rather than the tangential velocity.
Virtual Physics
Ladybug motion in 2d.
In this simulation, you experiment with the position, velocity, and acceleration of a ladybug in circular and elliptical motion. Switch the type of motion from linear to circular and observe the velocity and acceleration vectors. Next, try elliptical motion and notice how the velocity and acceleration vectors differ from those in circular motion.
Grasp Check
In uniform circular motion, what is the angle between the acceleration and the velocity? What type of acceleration does a body experience in the uniform circular motion?
- The angle between acceleration and velocity is 0°, and the body experiences linear acceleration.
- The angle between acceleration and velocity is 0°, and the body experiences centripetal acceleration.
- The angle between acceleration and velocity is 90°, and the body experiences linear acceleration.
- The angle between acceleration and velocity is 90°, and the body experiences centripetal acceleration.
Centripetal Force
[BL] [OL] [AL] Using the same demonstration as before, ask students to predict the relationships between the quantities of angular velocity, centripetal acceleration, mass, centripetal force. Invite students to experiment by using various lengths of string and different weights.
Because an object in uniform circular motion undergoes acceleration (by changing the direction of motion but not the speed), we know from Newton’s second law of motion that there must be a net external force acting on the object. Since the magnitude of the acceleration is constant, so is the magnitude of the net force, and since the acceleration points toward the center of the rotation, so does the net force.
Any force or combination of forces can cause a centripetal acceleration. Just a few examples are the tension in the rope on a tether ball, the force of Earth’s gravity on the Moon, the friction between a road and the tires of a car as it goes around a curve, or the normal force of a roller coaster track on the cart during a loop-the-loop.
The component of any net force that causes circular motion is called a centripetal force . When the net force is equal to the centripetal force, and its magnitude is constant, uniform circular motion results. The direction of a centripetal force is toward the center of rotation, the same as for centripetal acceleration. According to Newton’s second law of motion, a net force causes the acceleration of mass according to F net = m a . For uniform circular motion, the acceleration is centripetal acceleration: a = a c . Therefore, the magnitude of centripetal force, F c , is F c = m a c F c = m a c .
By using the two different forms of the equation for the magnitude of centripetal acceleration, a c = v 2 / r a c = v 2 / r and a c = r ω 2 a c = r ω 2 , we get two expressions involving the magnitude of the centripetal force F c F c . The first expression is in terms of tangential speed, the second is in terms of angular speed: F c = m v 2 r F c = m v 2 r and F c = m r ω 2 F c = m r ω 2 .
Both forms of the equation depend on mass, velocity, and the radius of the circular path. You may use whichever expression for centripetal force is more convenient. Newton’s second law also states that the object will accelerate in the same direction as the net force. By definition, the centripetal force is directed towards the center of rotation, so the object will also accelerate towards the center. A straight line drawn from the circular path to the center of the circle will always be perpendicular to the tangential velocity. Note that, if you solve the first expression for r , you get
From this expression, we see that, for a given mass and velocity, a large centripetal force causes a small radius of curvature—that is, a tight curve.
Watch Physics
Centripetal force and acceleration intuition.
This video explains why centripetal force, when it is equal to the net force and has constant magnitude, creates centripetal acceleration and uniform circular motion.
Misconception Alert
Some students might be confused between centripetal force and centrifugal force. Centrifugal force is not a real force but the result of an accelerating reference frame, such as a turning car or the spinning Earth. Centrifugal force refers to a fictional center fleeing force.
- The yoyo will fly inward in the direction of the centripetal force.
- The yoyo will fly outward in the direction of the centripetal force.
- The yoyo will fly to the left in the direction of the tangential velocity.
- The yoyo will fly to the right in the direction of the tangential velocity.
Solving Centripetal Acceleration and Centripetal Force Problems
To get a feel for the typical magnitudes of centripetal acceleration, we’ll do a lab estimating the centripetal acceleration of a tennis racket and then, in our first Worked Example, compare the centripetal acceleration of a car rounding a curve to gravitational acceleration. For the second Worked Example, we’ll calculate the force required to make a car round a curve.
Estimating Centripetal Acceleration
In this activity, you will measure the swing of a golf club or tennis racket to estimate the centripetal acceleration of the end of the club or racket. You may choose to do this in slow motion. Recall that the equation for centripetal acceleration is a c = v 2 r a c = v 2 r or a c = r ω 2 a c = r ω 2 .
- One tennis racket or golf club
- One ruler or tape measure
- Work with a partner. Stand a safe distance away from your partner as he or she swings the golf club or tennis racket.
- Describe the motion of the swing—is this uniform circular motion? Why or why not?
- Try to get the swing as close to uniform circular motion as possible. What adjustments did your partner need to make?
- Measure the radius of curvature. What did you physically measure?
- By using the timer, find either the linear or angular velocity, depending on which equation you decide to use.
- What is the approximate centripetal acceleration based on these measurements? How accurate do you think they are? Why? How might you and your partner make these measurements more accurate?
The swing of the golf club or racket can be made very close to uniform circular motion. For this, the person would have to move it at a constant speed, without bending their arm. The length of the arm plus the length of the club or racket is the radius of curvature. Accuracy of measurements of angular velocity and angular acceleration will depend on resolution of the timer used and human observational error. The swing of the golf club or racket can be made very close to uniform circular motion. For this, the person would have to move it at a constant speed, without bending their arm. The length of the arm plus the length of the club or racket is the radius of curvature. Accuracy of measurements of angular velocity and angular acceleration will depend on resolution of the timer used and human observational error.
Was it more useful to use the equation a c = v 2 r a c = v 2 r or a c = r ω 2 a c = r ω 2 in this activity? Why?
- It should be simpler to use a c = r ω 2 a c = r ω 2 because measuring angular velocity through observation would be easier.
- It should be simpler to use a c = v 2 r a c = v 2 r because measuring tangential velocity through observation would be easier.
- It should be simpler to use a c = r ω 2 a c = r ω 2 because measuring angular velocity through observation would be difficult.
- It should be simpler to use a c = v 2 r a c = v 2 r because measuring tangential velocity through observation would be difficult.
Worked Example
Comparing centripetal acceleration of a car rounding a curve with acceleration due to gravity.
A car follows a curve of radius 500 m at a speed of 25.0 m/s (about 90 km/h). What is the magnitude of the car’s centripetal acceleration? Compare the centripetal acceleration for this fairly gentle curve taken at highway speed with acceleration due to gravity ( g ).
Because linear rather than angular speed is given, it is most convenient to use the expression a c = v 2 r a c = v 2 r to find the magnitude of the centripetal acceleration.
Entering the given values of v = 25.0 m/s and r = 500 m into the expression for a c gives
To compare this with the acceleration due to gravity ( g = 9.80 m/s 2 ), we take the ratio a c / g = ( 1.25 m/s 2 ) / ( 9.80 m/s 2 ) = 0.128 a c / g = ( 1.25 m/s 2 ) / ( 9.80 m/s 2 ) = 0.128 . Therefore, a c = 0.128 g a c = 0.128 g , which means that the centripetal acceleration is about one tenth the acceleration due to gravity.
Frictional Force on Car Tires Rounding a Curve
- Calculate the centripetal force exerted on a 900 kg car that rounds a 600-m-radius curve on horizontal ground at 25.0 m/s.
- Static friction prevents the car from slipping. Find the magnitude of the frictional force between the tires and the road that allows the car to round the curve without sliding off in a straight line.
- If the car would slip if it were to be traveling any faster, what is the coefficient of static friction between the tires and the road? Could we conclude anything about the coefficient of static friction if we did not know whether the car could round the curve any faster without slipping?
Strategy and Solution for (a)
We know that F c = m v 2 r F c = m v 2 r . Therefore,
Strategy and Solution for (b)
The image above shows the forces acting on the car while rounding the curve. In this diagram, the car is traveling into the page as shown and is turning to the left. Friction acts toward the left, accelerating the car toward the center of the curve. Because friction is the only horizontal force acting on the car, it provides all of the centripetal force in this case. Therefore, the force of friction is the centripetal force in this situation and points toward the center of the curve.
Strategy and Solution for (c)
If the car is about to slip, the static friction is at its maximum value and f = μ s N = μ s m g f = μ s N = μ s m g . Solving for μ s μ s , we get μ s = 938 900 × 9.8 = 0.11 μ s = 938 900 × 9.8 = 0.11 . Regardless of whether we know the maximum allowable speed for rounding the curve, we can conclude this is a minimum value for the coefficient.
Since we found the force of friction in part (b), we could also solve for the coefficient of friction, since f = μ s N = μ s m g f = μ s N = μ s m g . The static friction is only equal to μ s N μ s N when it is at the maximum possible value. If the car could go faster, the friction at the given speed would still be the same as we calculated, but the coefficient of static friction would be larger.
Practice Problems
Calculate the centripetal acceleration of an object following a path with a radius of a curvature of 0.2 m and at an angular velocity of 5 rad/s.
Check Your Understanding
- Uniform circular motion is when an object accelerates on a circular path at a constantly increasing velocity.
- Uniform circular motion is when an object travels on a circular path at a variable acceleration.
- Uniform circular motion is when an object travels on a circular path at a constant speed.
- Uniform circular motion is when an object travels on a circular path at a variable speed.
Which of the following is centripetal acceleration?
- The acceleration of an object moving in a circular path and directed radially toward the center of the circular orbit
- The acceleration of an object moving in a circular path and directed tangentially along the circular path
- The acceleration of an object moving in a linear path and directed in the direction of motion of the object
- The acceleration of an object moving in a linear path and directed in the direction opposite to the motion of the object
- Yes, the object is accelerating, so a net force must be acting on it.
- Yes, because there is no acceleration.
- No, because there is acceleration.
- No, because there is no acceleration.
Identify two examples of forces that can cause centripetal acceleration.
- The force of Earth’s gravity on the moon and the normal force
- The force of Earth’s gravity on the moon and the tension in the rope on an orbiting tetherball
- The normal force and the force of friction acting on a moving car
- The normal force and the tension in the rope on a tetherball
Use the Check Your Understanding questions to assess whether students master the learning objectives of this section. If students are struggling with a specific objective, the formative assessment will help identify which objective is causing the problem and direct students to the relevant content.
As an Amazon Associate we earn from qualifying purchases.
This book may not be used in the training of large language models or otherwise be ingested into large language models or generative AI offerings without OpenStax's permission.
Want to cite, share, or modify this book? This book uses the Creative Commons Attribution License and you must attribute Texas Education Agency (TEA). The original material is available at: https://www.texasgateway.org/book/tea-physics . Changes were made to the original material, including updates to art, structure, and other content updates.
Access for free at https://openstax.org/books/physics/pages/1-introduction
- Authors: Paul Peter Urone, Roger Hinrichs
- Publisher/website: OpenStax
- Book title: Physics
- Publication date: Mar 26, 2020
- Location: Houston, Texas
- Book URL: https://openstax.org/books/physics/pages/1-introduction
- Section URL: https://openstax.org/books/physics/pages/6-2-uniform-circular-motion
© Jan 19, 2024 Texas Education Agency (TEA). The OpenStax name, OpenStax logo, OpenStax book covers, OpenStax CNX name, and OpenStax CNX logo are not subject to the Creative Commons license and may not be reproduced without the prior and express written consent of Rice University.
Investigating Circular Motion ( OCR A Level Physics )
Revision note.
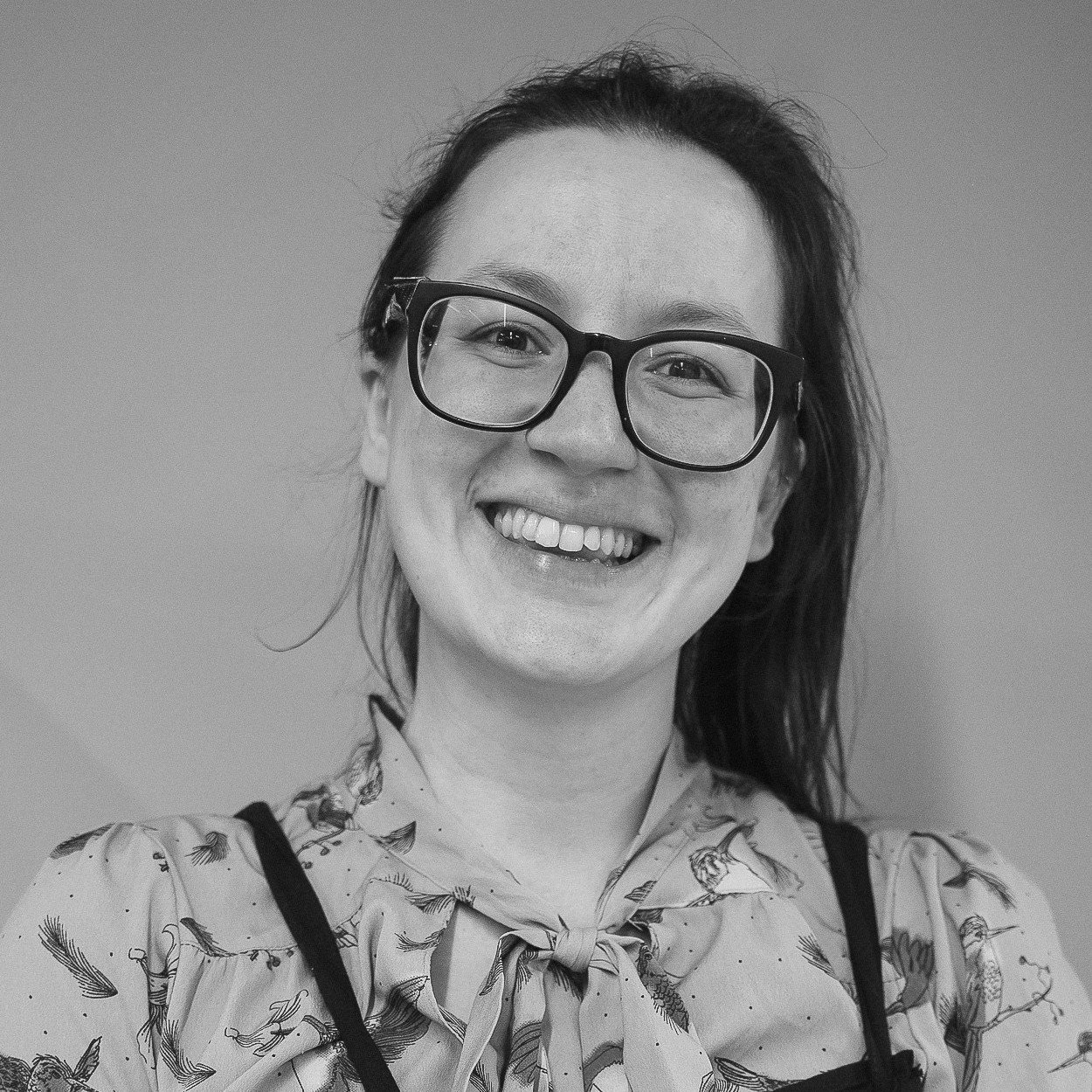

Investigating Circular Motion
Equipment & method.
- Tie a bung of mass m, to a piece of string, which sits horizontally
- Thread it though a glass tube and a paper clip, which sits vertically
- At the other end of the string a heavier mass, M is suspended vertically
- This provides the centripetal force, F = Mg when the tension in the string is constant
- The time taken for several rotations is recorded and repeated to remove any random errors
- The masses in the experimental set up are changed before the experiment is repeated again
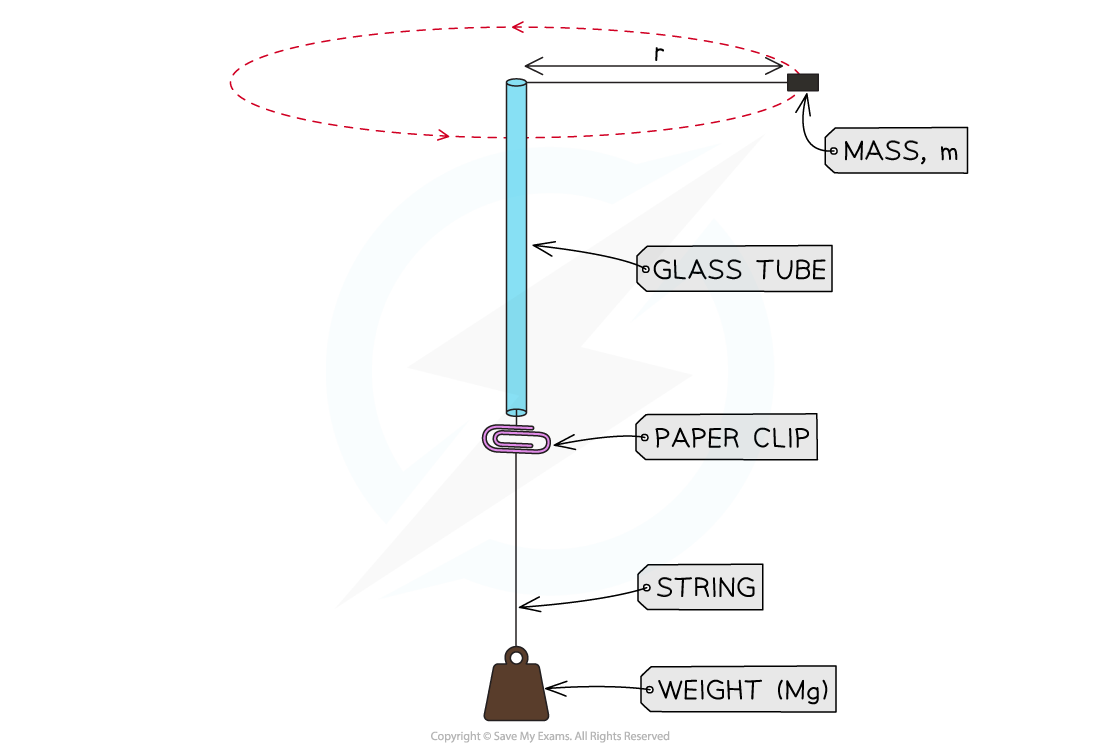
Explanation
- When the force it provides is equal to the centripetal force , Mg
- This is the centripetal force required to make the bung travel in a circular path
- The weight , and hence the centripetal force , required for different masses, radii and speeds can be investigated
- The tension in the string
- The weight of the bung downwards
- If the centripetal force required is greater than its weight then the suspended mass moves upwards
- The paperclip will move accordingly to make this movement clearer
- As the bung moves around the circle, the direction of the tension will change continuously
- This is because the direction of the weight of the bung never changes, so the resultant force will vary depending on the position of the bung in the circle
- At the bottom of the circle, the tension must overcome the weight, this can be written as:

- As a result, the acceleration, and hence, the speed of the bung will be slower at the top
- At the top of the circle, the tension and weight act in the same direction, this can be written as:

- As a result, the acceleration, and hence, the speed of the bung will be faster at the bottom
You've read 0 of your 0 free revision notes
Get unlimited access.
to absolutely everything:
- Downloadable PDFs
- Unlimited Revision Notes
- Topic Questions
- Past Papers
- Model Answers
- Videos (Maths and Science)
Join the 100,000 + Students that ❤️ Save My Exams
the (exam) results speak for themselves:
Did this page help you?
Author: Katie M
Katie has always been passionate about the sciences, and completed a degree in Astrophysics at Sheffield University. She decided that she wanted to inspire other young people, so moved to Bristol to complete a PGCE in Secondary Science. She particularly loves creating fun and absorbing materials to help students achieve their exam potential.
Uniform Circular Motion Lab
This experiment investigates how the force necessary to maintain a body in uniform circular motion (i.e. in a circle of constant radius and with a constant speed) is related to the speed of the object experiencing UCM. You should note that there are other possibilities for the independent variable. We are keeping all of the possible independent variables fixed save the applied force.
Your apparatus, shown in figure 1, consists of a glass tube, some string, a rubber stopper, and an alligator clip. The force that keeps the rubber stopper traveling in a circle at constant speed is supplied by the string. The source of the force is gravity pulling down on a mass attached to the lower end of the string. If we ignore the friction of the string on the glass tube, the force downward on the mass due to gravity is the same as the force inward on the stopper. Hence the force is M hanging g and the velocity can be calculated by measuring the time for thirty complete circles, calculating the distance traveled (30 times the circumference), and then the average speed.
- Measure the mass of your stopper. (You won't need it until the end, but measuring it now makes sure you don't forget to do it.)
- Thread the string through the tube.
- Attach the stopper to one end and 150 g of mass to the lower end.
- Stretch the apparatus out on your lab table and position the stopper so that the distance between the middle of the stopper and the top of the tube is 50 cm.
- Attach the alligator clip about 2 cm below the bottom of the tube.
- Holding the tube in one hand, start swinging the stopper slowly in a circle above your head. As you swing faster, the clip will rise. This happens because the suspended mass can't supply enough force to keep the stopper from moving out. Thus, the radius increases and the force needed to maintain UCM will then decrease (due to the larger radius the needed acceleration is smaller). After a little practice, you should be able to maintain the alligator clip about 2 cm below the bottom of the tube.
- Have a partner start a stopwatch and time the amount of time needed for thirty complete revolutions. You should concentrate on trying to keep the alligator clip in a steady position 2 cm below the bottom of the tube.
- Once the time has been measured, record your data in a table (similar to the example below) and proceed on to other masses.
- You should acquire additional data for hanging masses of: 20, 30, 50, 100, 200, 250, and 280 grams.
For each case, we know the radius of the circle is equal to the value set earlier, and that the applied force is the weight of the hanging mass. Calculate the force applied, and the resulting velocity for each case and complete a table like the one shown in your notebook.
(kg) |
|
| (Nt.) |
Once your table is complete, plot a graph of velocity vs. applied force. What is the shape of the graph? If it is straight, draw in the best fit straight line and determine the equation for the best fit line. If the graph is not straight, use its shape to figure out how to re-plot the graph in such a manner as to obtain a straight (or straighter) line. Draw in the best fit line for this graph and determine the equation which describes the line.
Use Newton's second law ( with the net force being the weight of the suspended mass) and your knowledge of circular motion to predict what the slope of your line should be. ( i.e. solve for v in terms of F net . Calculate this theoretical slope. Compare this theoretical slope with the slope that you got from your best fit line. How well do they agree? Make a verbal comparison and also calculate the percentage difference between the experimental and theoretical slopes. Which slope is larger? What are some possible experimental reasons that the two might not agree? For one of the possible sources of errors (i.e experimental uncertainty not mistakes), think about whether it would tend to increase or to decrease the measured slope? Explain your reasoning.
Barney Taylor Physics Dept. Miami University - Hamilton 1601 University Blvd., Hamilton, OH 45011 (513) 785-3016 [email protected]
last modified Aug, 2013
Remember Me
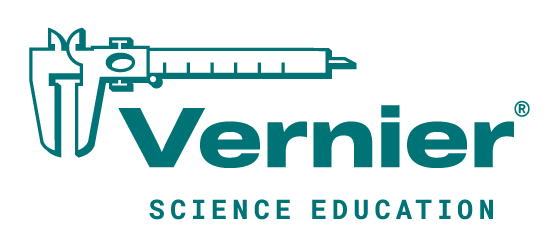
Shop Experiment Circular Motion Experiments
Circular motion.
Experiment #8 from Physics Explorations and Projects
Introduction
The goal of this activity is for students to determine the relationship between the (angular or linear) velocity, radius, and mass on the centripetal force or acceleration necessary to keep an object moving in a circular path.
In the Preliminary Observations, students will observe an object that is swung on a string in a circular path. Students then explore force (or acceleration) and circular motion using a method of their choosing, though instructors may provide a limited number of choices.
During the subsequent inquiry process, students may use a variety of tools ranging from a mass on a string to the Centripetal Force Apparatus. This activity can also be done virtually using Pivot Interactives.
Students should finish the activity having evaluated data graphically and developed an expression relating a center-pointed (centripetal) net force to the properties of circular motion.
- Design and perform an investigation.
- Draw a conclusion from evidence.
- Understand that the centripetal force acting on (or the centripetal acceleration of) an object moving in a circular pattern is governed by one of two relationships
Sensors and Equipment
This experiment features the following sensors and equipment. Additional equipment may be required.
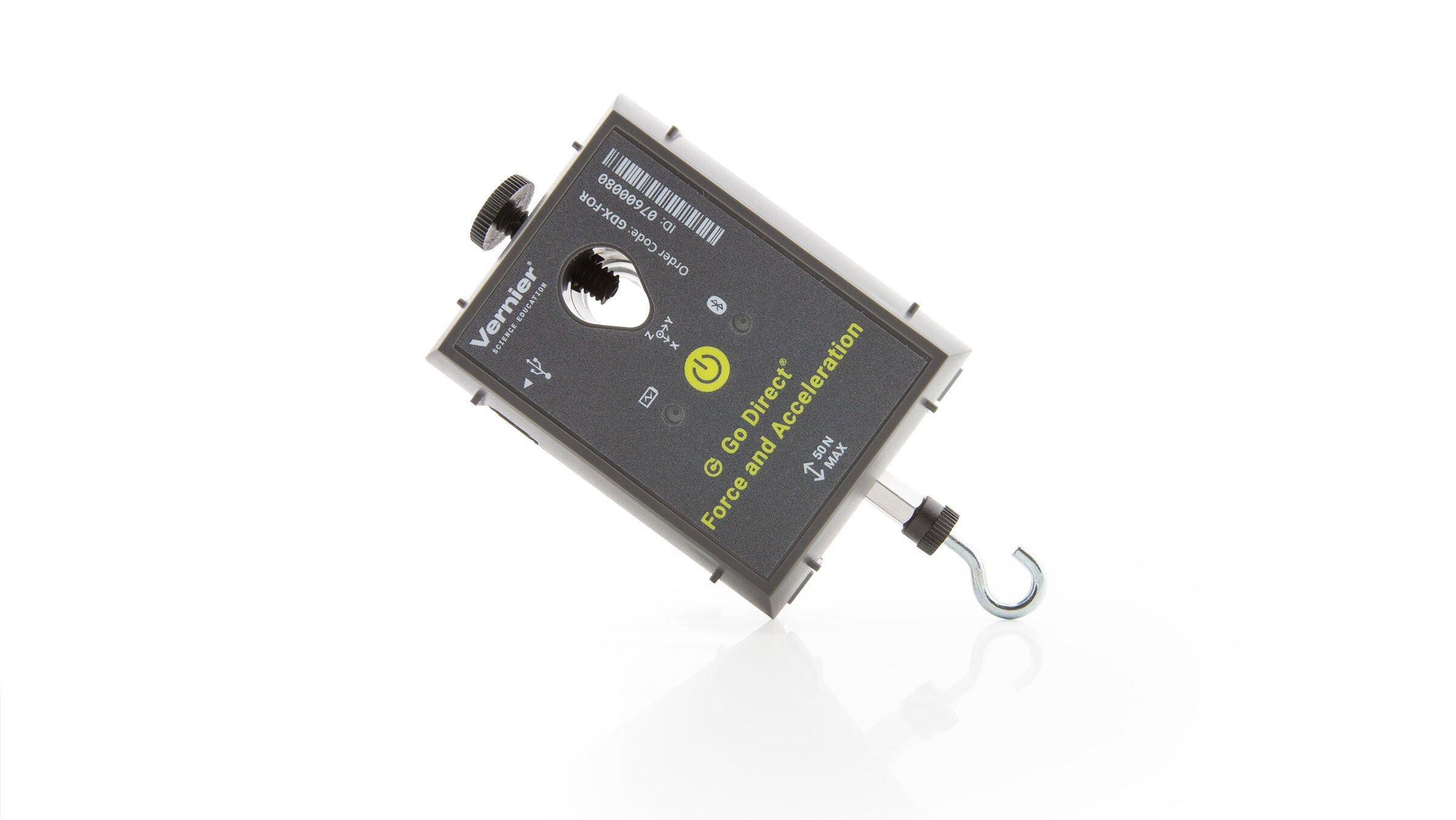
Correlations
Teaching to an educational standard? This experiment supports the standards below.
Ready to Experiment?
Ask an expert.
Get answers to your questions about how to teach this experiment with our support team.
- Call toll-free: 888-837-6437
- Chat with Us
- Email [email protected]
Purchase the Lab Book
This experiment is #8 of Physics Explorations and Projects . The experiment in the book includes student instructions as well as instructor information for set up, helpful hints, and sample graphs and data.
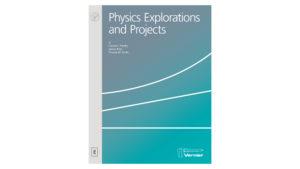
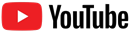
- TPC and eLearning
- What's NEW at TPC?
- Read Watch Interact
- Practice Review Test
- Teacher-Tools
- Request a Demo
- Get A Quote
- Subscription Selection
- Seat Calculator
- Ad Free Account
- Edit Profile Settings
- Metric Conversions Questions
- Metric System Questions
- Metric Estimation Questions
- Significant Digits Questions
- Proportional Reasoning
- Acceleration
- Distance-Displacement
- Dots and Graphs
- Graph That Motion
- Match That Graph
- Name That Motion
- Motion Diagrams
- Pos'n Time Graphs Numerical
- Pos'n Time Graphs Conceptual
- Up And Down - Questions
- Balanced vs. Unbalanced Forces
- Change of State
- Force and Motion
- Mass and Weight
- Match That Free-Body Diagram
- Net Force (and Acceleration) Ranking Tasks
- Newton's Second Law
- Normal Force Card Sort
- Recognizing Forces
- Air Resistance and Skydiving
- Solve It! with Newton's Second Law
- Which One Doesn't Belong?
- Component Addition Questions
- Head-to-Tail Vector Addition
- Projectile Mathematics
- Trajectory - Angle Launched Projectiles
- Trajectory - Horizontally Launched Projectiles
- Vector Addition
- Vector Direction
- Which One Doesn't Belong? Projectile Motion
- Forces in 2-Dimensions
- Being Impulsive About Momentum
- Explosions - Law Breakers
- Hit and Stick Collisions - Law Breakers
- Case Studies: Impulse and Force
- Impulse-Momentum Change Table
- Keeping Track of Momentum - Hit and Stick
- Keeping Track of Momentum - Hit and Bounce
- What's Up (and Down) with KE and PE?
- Energy Conservation Questions
- Energy Dissipation Questions
- Energy Ranking Tasks
- LOL Charts (a.k.a., Energy Bar Charts)
- Match That Bar Chart
- Words and Charts Questions
- Name That Energy
- Stepping Up with PE and KE Questions
- Case Studies - Circular Motion
- Circular Logic
- Forces and Free-Body Diagrams in Circular Motion
- Gravitational Field Strength
- Universal Gravitation
- Angular Position and Displacement
- Linear and Angular Velocity
- Angular Acceleration
- Rotational Inertia
- Balanced vs. Unbalanced Torques
- Getting a Handle on Torque
- Torque-ing About Rotation
- Properties of Matter
- Fluid Pressure
- Buoyant Force
- Sinking, Floating, and Hanging
- Pascal's Principle
- Flow Velocity
- Bernoulli's Principle
- Balloon Interactions
- Charge and Charging
- Charge Interactions
- Charging by Induction
- Conductors and Insulators
- Coulombs Law
- Electric Field
- Electric Field Intensity
- Polarization
- Case Studies: Electric Power
- Know Your Potential
- Light Bulb Anatomy
- I = ∆V/R Equations as a Guide to Thinking
- Parallel Circuits - ∆V = I•R Calculations
- Resistance Ranking Tasks
- Series Circuits - ∆V = I•R Calculations
- Series vs. Parallel Circuits
- Equivalent Resistance
- Period and Frequency of a Pendulum
- Pendulum Motion: Velocity and Force
- Energy of a Pendulum
- Period and Frequency of a Mass on a Spring
- Horizontal Springs: Velocity and Force
- Vertical Springs: Velocity and Force
- Energy of a Mass on a Spring
- Decibel Scale
- Frequency and Period
- Closed-End Air Columns
- Name That Harmonic: Strings
- Rocking the Boat
- Wave Basics
- Matching Pairs: Wave Characteristics
- Wave Interference
- Waves - Case Studies
- Color Addition and Subtraction
- Color Filters
- If This, Then That: Color Subtraction
- Light Intensity
- Color Pigments
- Converging Lenses
- Curved Mirror Images
- Law of Reflection
- Refraction and Lenses
- Total Internal Reflection
- Who Can See Who?
- Formulas and Atom Counting
- Lab Equipment
- Atomic Models
- Bond Polarity
- Entropy Questions
- Cell Voltage Questions
- Heat of Formation Questions
- Reduction Potential Questions
- Oxidation States Questions
- Measuring the Quantity of Heat
- Hess's Law
- Oxidation-Reduction Questions
- Galvanic Cells Questions
- Thermal Stoichiometry
- Molecular Polarity
- Quantum Mechanics
- Balancing Chemical Equations
- Bronsted-Lowry Model of Acids and Bases
- Classification of Matter
- Collision Model of Reaction Rates
- Density Ranking Tasks
- Dissociation Reactions
- Complete Electron Configurations
- Elemental Measures
- Enthalpy Change Questions
- Equilibrium Concept
- Equilibrium Constant Expression
- Equilibrium Calculations - Questions
- Equilibrium ICE Table
- Intermolecular Forces Questions
- Ionic Bonding
- Lewis Electron Dot Structures
- Limiting Reactants
- Line Spectra Questions
- Mass Stoichiometry
- Measurement and Numbers
- Metals, Nonmetals, and Metalloids
- Metric Estimations
- Metric System
- Molarity Ranking Tasks
- Mole Conversions
- Name That Element
- Names to Formulas
- Names to Formulas 2
- Nuclear Decay
- Particles, Words, and Formulas
- Periodic Trends
- Precipitation Reactions and Net Ionic Equations
- Pressure Concepts
- Pressure-Temperature Gas Law
- Pressure-Volume Gas Law
- Chemical Reaction Types
- Significant Digits and Measurement
- States Of Matter Exercise
- Stoichiometry Law Breakers
- Stoichiometry - Math Relationships
- Subatomic Particles
- Spontaneity and Driving Forces
- Gibbs Free Energy
- Volume-Temperature Gas Law
- Acid-Base Properties
- Energy and Chemical Reactions
- Chemical and Physical Properties
- Valence Shell Electron Pair Repulsion Theory
- Writing Balanced Chemical Equations
- Mission CG1
- Mission CG10
- Mission CG2
- Mission CG3
- Mission CG4
- Mission CG5
- Mission CG6
- Mission CG7
- Mission CG8
- Mission CG9
- Mission EC1
- Mission EC10
- Mission EC11
- Mission EC12
- Mission EC2
- Mission EC3
- Mission EC4
- Mission EC5
- Mission EC6
- Mission EC7
- Mission EC8
- Mission EC9
- Mission RL1
- Mission RL2
- Mission RL3
- Mission RL4
- Mission RL5
- Mission RL6
- Mission KG7
- Mission RL8
- Mission KG9
- Mission RL10
- Mission RL11
- Mission RM1
- Mission RM2
- Mission RM3
- Mission RM4
- Mission RM5
- Mission RM6
- Mission RM8
- Mission RM10
- Mission LC1
- Mission RM11
- Mission LC2
- Mission LC3
- Mission LC4
- Mission LC5
- Mission LC6
- Mission LC8
- Mission SM1
- Mission SM2
- Mission SM3
- Mission SM4
- Mission SM5
- Mission SM6
- Mission SM8
- Mission SM10
- Mission KG10
- Mission SM11
- Mission KG2
- Mission KG3
- Mission KG4
- Mission KG5
- Mission KG6
- Mission KG8
- Mission KG11
- Mission F2D1
- Mission F2D2
- Mission F2D3
- Mission F2D4
- Mission F2D5
- Mission F2D6
- Mission KC1
- Mission KC2
- Mission KC3
- Mission KC4
- Mission KC5
- Mission KC6
- Mission KC7
- Mission KC8
- Mission AAA
- Mission SM9
- Mission LC7
- Mission LC9
- Mission NL1
- Mission NL2
- Mission NL3
- Mission NL4
- Mission NL5
- Mission NL6
- Mission NL7
- Mission NL8
- Mission NL9
- Mission NL10
- Mission NL11
- Mission NL12
- Mission MC1
- Mission MC10
- Mission MC2
- Mission MC3
- Mission MC4
- Mission MC5
- Mission MC6
- Mission MC7
- Mission MC8
- Mission MC9
- Mission RM7
- Mission RM9
- Mission RL7
- Mission RL9
- Mission SM7
- Mission SE1
- Mission SE10
- Mission SE11
- Mission SE12
- Mission SE2
- Mission SE3
- Mission SE4
- Mission SE5
- Mission SE6
- Mission SE7
- Mission SE8
- Mission SE9
- Mission VP1
- Mission VP10
- Mission VP2
- Mission VP3
- Mission VP4
- Mission VP5
- Mission VP6
- Mission VP7
- Mission VP8
- Mission VP9
- Mission WM1
- Mission WM2
- Mission WM3
- Mission WM4
- Mission WM5
- Mission WM6
- Mission WM7
- Mission WM8
- Mission WE1
- Mission WE10
- Mission WE2
- Mission WE3
- Mission WE4
- Mission WE5
- Mission WE6
- Mission WE7
- Mission WE8
- Mission WE9
- Vector Walk Interactive
- Name That Motion Interactive
- Kinematic Graphing 1 Concept Checker
- Kinematic Graphing 2 Concept Checker
- Graph That Motion Interactive
- Two Stage Rocket Interactive
- Rocket Sled Concept Checker
- Force Concept Checker
- Free-Body Diagrams Concept Checker
- Free-Body Diagrams The Sequel Concept Checker
- Skydiving Concept Checker
- Elevator Ride Concept Checker
- Vector Addition Concept Checker
- Vector Walk in Two Dimensions Interactive
- Name That Vector Interactive
- River Boat Simulator Concept Checker
- Projectile Simulator 2 Concept Checker
- Projectile Simulator 3 Concept Checker
- Hit the Target Interactive
- Turd the Target 1 Interactive
- Turd the Target 2 Interactive
- Balance It Interactive
- Go For The Gold Interactive
- Egg Drop Concept Checker
- Fish Catch Concept Checker
- Exploding Carts Concept Checker
- Collision Carts - Inelastic Collisions Concept Checker
- Its All Uphill Concept Checker
- Stopping Distance Concept Checker
- Chart That Motion Interactive
- Roller Coaster Model Concept Checker
- Uniform Circular Motion Concept Checker
- Horizontal Circle Simulation Concept Checker
- Vertical Circle Simulation Concept Checker
- Race Track Concept Checker
- Gravitational Fields Concept Checker
- Orbital Motion Concept Checker
- Angular Acceleration Concept Checker
- Balance Beam Concept Checker
- Torque Balancer Concept Checker
- Aluminum Can Polarization Concept Checker
- Charging Concept Checker
- Name That Charge Simulation
- Coulomb's Law Concept Checker
- Electric Field Lines Concept Checker
- Put the Charge in the Goal Concept Checker
- Circuit Builder Concept Checker (Series Circuits)
- Circuit Builder Concept Checker (Parallel Circuits)
- Circuit Builder Concept Checker (∆V-I-R)
- Circuit Builder Concept Checker (Voltage Drop)
- Equivalent Resistance Interactive
- Pendulum Motion Simulation Concept Checker
- Mass on a Spring Simulation Concept Checker
- Particle Wave Simulation Concept Checker
- Boundary Behavior Simulation Concept Checker
- Slinky Wave Simulator Concept Checker
- Simple Wave Simulator Concept Checker
- Wave Addition Simulation Concept Checker
- Standing Wave Maker Simulation Concept Checker
- Color Addition Concept Checker
- Painting With CMY Concept Checker
- Stage Lighting Concept Checker
- Filtering Away Concept Checker
- InterferencePatterns Concept Checker
- Young's Experiment Interactive
- Plane Mirror Images Interactive
- Who Can See Who Concept Checker
- Optics Bench (Mirrors) Concept Checker
- Name That Image (Mirrors) Interactive
- Refraction Concept Checker
- Total Internal Reflection Concept Checker
- Optics Bench (Lenses) Concept Checker
- Kinematics Preview
- Velocity Time Graphs Preview
- Moving Cart on an Inclined Plane Preview
- Stopping Distance Preview
- Cart, Bricks, and Bands Preview
- Fan Cart Study Preview
- Friction Preview
- Coffee Filter Lab Preview
- Friction, Speed, and Stopping Distance Preview
- Up and Down Preview
- Projectile Range Preview
- Ballistics Preview
- Juggling Preview
- Marshmallow Launcher Preview
- Air Bag Safety Preview
- Colliding Carts Preview
- Collisions Preview
- Engineering Safer Helmets Preview
- Push the Plow Preview
- Its All Uphill Preview
- Energy on an Incline Preview
- Modeling Roller Coasters Preview
- Hot Wheels Stopping Distance Preview
- Ball Bat Collision Preview
- Energy in Fields Preview
- Weightlessness Training Preview
- Roller Coaster Loops Preview
- Universal Gravitation Preview
- Keplers Laws Preview
- Kepler's Third Law Preview
- Charge Interactions Preview
- Sticky Tape Experiments Preview
- Wire Gauge Preview
- Voltage, Current, and Resistance Preview
- Light Bulb Resistance Preview
- Series and Parallel Circuits Preview
- Thermal Equilibrium Preview
- Linear Expansion Preview
- Heating Curves Preview
- Electricity and Magnetism - Part 1 Preview
- Electricity and Magnetism - Part 2 Preview
- Vibrating Mass on a Spring Preview
- Period of a Pendulum Preview
- Wave Speed Preview
- Slinky-Experiments Preview
- Standing Waves in a Rope Preview
- Sound as a Pressure Wave Preview
- DeciBel Scale Preview
- DeciBels, Phons, and Sones Preview
- Sound of Music Preview
- Shedding Light on Light Bulbs Preview
- Models of Light Preview
- Electromagnetic Radiation Preview
- Electromagnetic Spectrum Preview
- EM Wave Communication Preview
- Digitized Data Preview
- Light Intensity Preview
- Concave Mirrors Preview
- Object Image Relations Preview
- Snells Law Preview
- Reflection vs. Transmission Preview
- Magnification Lab Preview
- Reactivity Preview
- Ions and the Periodic Table Preview
- Periodic Trends Preview
- Chemical Reactions Preview
- Intermolecular Forces Preview
- Melting Points and Boiling Points Preview
- Bond Energy and Reactions Preview
- Reaction Rates Preview
- Ammonia Factory Preview
- Stoichiometry Preview
- Nuclear Chemistry Preview
- Gaining Teacher Access
- Task Tracker Directions
- Conceptual Physics Course
- On-Level Physics Course
- Honors Physics Course
- Chemistry Concept Builders
- All Chemistry Resources
- Users Voice
- Tasks and Classes
- Webinars and Trainings
- Subscription
- Subscription Locator
- 1-D Kinematics
- Newton's Laws
- Vectors - Motion and Forces in Two Dimensions
- Momentum and Its Conservation
- Work and Energy
- Circular Motion and Satellite Motion
- Thermal Physics
- Static Electricity
- Electric Circuits
- Vibrations and Waves
- Sound Waves and Music
- Light and Color
- Reflection and Mirrors
- About the Physics Interactives
- Task Tracker
- Usage Policy
- Newtons Laws
- Vectors and Projectiles
- Forces in 2D
- Momentum and Collisions
- Circular and Satellite Motion
- Balance and Rotation
- Electromagnetism
- Waves and Sound
- Atomic Physics
- Forces in Two Dimensions
- Work, Energy, and Power
- Circular Motion and Gravitation
- Sound Waves
- 1-Dimensional Kinematics
- Circular, Satellite, and Rotational Motion
- Einstein's Theory of Special Relativity
- Waves, Sound and Light
- QuickTime Movies
- About the Concept Builders
- Pricing For Schools
- Directions for Version 2
- Measurement and Units
- Relationships and Graphs
- Rotation and Balance
- Vibrational Motion
- Reflection and Refraction
- Teacher Accounts
- Kinematic Concepts
- Kinematic Graphing
- Wave Motion
- Sound and Music
- About CalcPad
- 1D Kinematics
- Vectors and Forces in 2D
- Simple Harmonic Motion
- Rotational Kinematics
- Rotation and Torque
- Rotational Dynamics
- Electric Fields, Potential, and Capacitance
- Transient RC Circuits
- Light Waves
- Units and Measurement
- Stoichiometry
- Molarity and Solutions
- Thermal Chemistry
- Acids and Bases
- Kinetics and Equilibrium
- Solution Equilibria
- Oxidation-Reduction
- Nuclear Chemistry
- Newton's Laws of Motion
- Work and Energy Packet
- Static Electricity Review
- NGSS Alignments
- 1D-Kinematics
- Projectiles
- Circular Motion
- Magnetism and Electromagnetism
- Graphing Practice
- About the ACT
- ACT Preparation
- For Teachers
- Other Resources
- Solutions Guide
- Solutions Guide Digital Download
- Motion in One Dimension
- Work, Energy and Power
- Algebra Based On-Level Physics
- Honors Physics
- Conceptual Physics
- Other Tools
- Frequently Asked Questions
- Purchasing the Download
- Purchasing the Digital Download
- About the NGSS Corner
- NGSS Search
- Force and Motion DCIs - High School
- Energy DCIs - High School
- Wave Applications DCIs - High School
- Force and Motion PEs - High School
- Energy PEs - High School
- Wave Applications PEs - High School
- Crosscutting Concepts
- The Practices
- Physics Topics
- NGSS Corner: Activity List
- NGSS Corner: Infographics
- About the Toolkits
- Position-Velocity-Acceleration
- Position-Time Graphs
- Velocity-Time Graphs
- Newton's First Law
- Newton's Second Law
- Newton's Third Law
- Terminal Velocity
- Projectile Motion
- Forces in 2 Dimensions
- Impulse and Momentum Change
- Momentum Conservation
- Work-Energy Fundamentals
- Work-Energy Relationship
- Roller Coaster Physics
- Satellite Motion
- Electric Fields
- Circuit Concepts
- Series Circuits
- Parallel Circuits
- Describing-Waves
- Wave Behavior Toolkit
- Standing Wave Patterns
- Resonating Air Columns
- Wave Model of Light
- Plane Mirrors
- Curved Mirrors
- Teacher Guide
- Using Lab Notebooks
- Current Electricity
- Light Waves and Color
- Reflection and Ray Model of Light
- Refraction and Ray Model of Light
- Teacher Resources
- Subscriptions

- Newton's Laws
- Einstein's Theory of Special Relativity
- About Concept Checkers
- School Pricing
- Newton's Laws of Motion
- Newton's First Law
- Newton's Third Law
Using the Interactive
Circular Motion: Mass on a String
Hsc physics syllabus.
analyse the forces acting on an object executing uniform circular motion in a variety of situations, for example:
Circular Motion Involving Tension Force
This video discusses the analysis of uniform circular motion scenarios involving a mass on a string or rope.
Understanding Circular Motion with a String
When an object is tethered to a string or rope and set into circular motion, like a ball swung in a circle, it's the tension in the string that provides the necessary centripetal force to keep the object moving in that circle.
Role of Tension in Circular Motion
Tension is a force exerted by a string or rope when it is stretched. In circular motion with a string:
Tension Force : Always pulls inward, towards the centre of the circular path. It contributes to the centripetal force, ensuring the object remains in its circular trajectory.
Centripetal Force : This isn’t a new or separate force. Instead, in the context of our spinning object, the tension in the string provides the centripetal force.
The relationship between tension and centripetal force depends on the angle the string or rope makes with either the vertical or horizontal axis.
When String is Horizontal
Consider a ball or mass attached to a string, undergoing uniform circular motion in a horizontal path as shown.

It is important to understand that this situation is only possible if the weight force of the mass is relatively negligible compared to the tension force. This is because the weight force is not balanced by any other forces, and would result in a net force acting downward on the mass, causing the string to create an angle with the horizontal axis.
In this case, the centripetal force is provided by the tension force:
$$F_{\text{centripetal}} = T$$
$$\frac{mv^2}{r} = T$$
This equation suggests that the magnitude of tension force depends on:
- Mass of the object : A heavier object requires more centripetal force, hence more tension, to keep it in a circular path.
- Radius of the circle : A larger circle or greater distance from the centre reduces the tension required, while a smaller circle increases it.
- Speed of the object : The faster the object moves, the greater the necessary centripetal force, and thus the greater the tension in the string.
In order to create such a circular path, the magnitude of tension needs to be substantially greater than the object's weight force. This can be achieved by increasing the object's speed, decreasing the length of the string (thus the radius), or using a smaller mass.
When String is at an Angle
Consider a ball or mass attached to a string, undergoing uniform circular motion in a horizontal path as shown. The string makes an angle θ with the vertical axis.

When the string or rope is at an angle, the direction of the tension force is also at an angle. This means we must resolve the tension force vector into its horizontal and vertical components.
$$T_x = T\sin{\theta}$$
$$T_y = T\cos{\theta}$$
In this case, the only force vector directed towards the centre of the circular path is the horizontal component of tension force. Therefore, the centripetal force is provided by the horizontal component of tension:
$$F_{\text{centripetal}} = T\sin{\theta}$$
$$\frac{mv^2}{r} = T\sin{\theta}$$
The vertical component of tension must balance the downward weight force of the mass if undergoes a horizontal circular motion.
$$mg = T\cos{\theta}$$
By dividing the two equations, we get:
$$\frac{v^2}{rg} = \tan{\theta}$$
Rearranging this equation, we can derive an expression for velocity:
$$v = \sqrt{rg\tan{\theta}}$$
Therefore, the velocity of the mass on a string in circular motion depends on
- radius of circular path
- acceleration due to gravity
- angle the string makes with the vertical axis; when angle increases, velocity increases
Vertical Circular Motion
Mass on a string or rope can be swung to create circular motion in a vertical orientation. In this instance, uniform circular motion can be achieved by ensuring that the centripetal force (net force) acting on an object remains constant.
It is important to understand that the direction and magnitude of different forces acting on the mass in a vertical circular motion change depending on its position.

For example, while the magnitude and direction of weight force remain unchanged, the direction and magnitude of tension change throughout the motion. At the top of the circular path, tension force acts downward. At the bottom of the circular path, tension force acts upward.
At the of the circular motion: |
`-(mv^2)/r=-mg-T_t`
`T_t=(mv^2)/r-mg` |
At the of the circular motion:
|
`(mv^2)/r=-mg+T_b`
`T_b=(mv^2)/r+mg` |
When an object attached to a rope is swung vertically, the tension in the rope changes throughout the motion. More tension force is needed at the bottom of the uniform circular motion compared to at the top because gravitational force acts in the opposite direction to centripetal force.
BACK TO MODULE 5: ADVANCED MECHANICS
- choosing a selection results in a full page refresh
- press the space key then arrow keys to make a selection

COMMENTS
restoring force on a string. In this experiment the tension in a string will act as the centripetal force on an object. THEORY: When a body moves with a constant speed in a circular path, it is said to move with uniform circular motion. Although the speed of the object is constant, the direction of the motion is continually changing.
In addition, the High School Physics Laboratory Manual addresses content in this section in the lab titled: Circular and Rotational Motion, as well as the following standards: (4) Science concepts. ... Invite students to experiment by using various lengths of string and different weights. Because an object in uniform circular motion undergoes ...
6. An open area to conduct the experiment. (Hallway or Classroom) Procedure: 1. Gather the materials to conduct the experiment. Choose an object and measure its mass. 2. Find a safe area to conduct the experiment. Safe = far enough away from other groups so that no students impede the motion of the object. 3. Securely tie the object onto a string.
Students can produce a table of results and in each case the force creating the circular motion, F, the tension in the string, is the weight of the washers. They compare this force with the calculated value of the force which is needed to perform a given orbit, mv 2 R. There are two difficulties which students commonly have with this experiment:
s the motion of a conical pendulum.College Board Big Ideas:4.D.1.1: The student is able to describe a representation and use it to analyze a situation in which several forces exerted on a rotating system of rigidly connected objects ch. e the angular velocity and angular momentum of the system. 4.D.1.2: The student is able to plan data ...
As we saw in Chapter 4, "uniform circular motion" is defined to be motion along a circle with constant speed. This may be a good time to review Section 4.4 for the kinematics of motion along a circle. In particular, for the uniform circular motion of an object around a circle of radius R, you should recall that: The velocity vector, →v.
As another example consider a ball, attached to a string and whirled in a circle as shown in Fig. 2a. The tension in the string applies the centripetal force to the ball, causing it to move in a circular path. The string pulls the ball toward the center of the circle while the ball pulls outward on the string and hence on your hand in accordance with Newton's third law of action and reaction.
Circular motion can be investigated using the following setup: Tie a bung of mass m, to a piece of string, which sits horizontally. Thread it though a glass tube and a paper clip, which sits vertically. At the other end of the string a heavier mass, M is suspended vertically. This provides the centripetal force, F = Mg when the tension in the ...
law used for circular motion is applied for a radial axis with the positive direction toward. the center of the circle (so the centripetal acceleration is positive) and in some cases to a. vertical y axis: ∑Fr = m ar ∑Fy = m ay where ar = ac = v2/r is the radial (centripetal) acceleration. III. Experiment 1—circular motion and the pendulum.
to. stretch the spring and bring the bob over the pointer byTthe equation F = W = M g.T3. Plot the centripetal force, F, as. a function of the centripetal acceleration, a, for each of the two plumb bob masses used. Plot the two sets of data on a single graph. (make sure to differentiate the points from the bare.
Experiment 5 Uniform Circular Motion. Whenever a body moves in a circular path, a force directed toward the center of the circle must act on the body to keep it moving in this path. That force is called centripetal force. The reaction, which is equal and opposite, is the pull of the body on the restraining medium and is called centrifugal force.
the actual phenomenon of circular motion that you will see in the lab. Centripetal Acceleration As you should be familiar with, acceleration is the rate of change of velocity. ... The radius is changed by moving the pin with which the string in looped around. Note: the radius is measured from the center "0" mark on the rotating platform and ...
A centripetal force is any force or combination of forces that causes an object to move in a circle with a constant speed. For this experiment, while the bob is rotating, there is only one force, the force due to a spring. Consequently, we can write the working equation for the centripetal force as. 4mrfi2.
Uniform Circular Motion Lab. This experiment investigates how the force necessary to maintain a body in uniform circular motion (i.e. in a circle of constant radius and with a constant speed) is related to the speed of the object experiencing UCM. ... Your apparatus, shown in figure 1, consists of a glass tube, some string, a rubber stopper ...
The goal of this activity is for students to determine the relationship between the (angular or linear) velocity, radius, and mass on the centripetal force or acceleration necessary to keep an object moving in a circular path. In the Preliminary Observations, students will observe an object that is swung on a string in a circular path. Students then explore force (or acceleration) and circular ...
The purpose behind this lab is for the student experimenters to investigate the centripetal motion and the force which keeps an object in circular motion. Students will continue to re ne their analysis skills. 2 Introduction The uniform circular motion of an object can be studied using this apparatus. FBD F s T W x y Side View of Circular ...
Lab: Circular Motion Student Guide Pre-Lab Information Purpose Conduct an investigation to explore the relationship between the centripetal force, mass, radius, and velocity of an object moving with uniform circular motion. ... the string to examine how the mass of the stopper affects its velocity. For the third part of the experiment, you will ...
Lab 4: Uniform Circular Motion SP211: Physics I Fall 2018 Dr. M.E. Jamer 1 Introduction Uniform circular motion occurs when an object goes around in a circle at constant speed. Note that although the object's velocity is constant in magnitude, it is not constant in direction, and thus the object is accelerating even though its speed is constant!
The Uniform Circular Motion Interactive is shown in the iFrame below. There is a small hot-spot in the lower-right corner of the iFrame. Dragging this hot-spot allows you to change the size of iFrame to whatever dimensions you prefer. Our Uniform Circular Motion simulation is now available with a Concept Checker. Do the simulation.
We begin with qualitative experiments that focus students' attention on the direction of the force causing a circular motion; later experiments are quantitative. ... Note the circular orbit before the string burns and the tangential motion afterwards. Puck D. The puck is connected to the suction cap with a spring, so an elliptic orbit ensues. ...
Part 2: An Alternate Method. In Part 1, an approximation is being made that the only force acting on the stopper is the tension in the string, and so the tension equals the centripetal force. In reality, gravity plays a role in the process as well. The string will actually be at an angle, as shown in Figure 1, rather than purely horizontal.
By dividing the two equations, we get: v2 rg = tanθ v 2 r g = tan. . θ. Rearranging this equation, we can derive an expression for velocity: v = √rgtanθ v = r g tan θ. Therefore, the velocity of the mass on a string in circular motion depends on. radius of circular path. acceleration due to gravity.
As an object travels in circular motion it is travelling at a constant speed but not at constant velocity. This is because as it travels in a circle, the object is always experiencing a change in direction. The acceleration of the object has a direction radiantly inwards towards the center of the circle. If not for the tension as the force ...